Hereditary Disease Foundation (HDF) conference 2024 – Day 3
Read our live tweet compilation from clinical trials and scientific research on Huntington’s disease from Day 3 of the 2024 HDF Milton Wexler Biennial Symposium #HDF2024
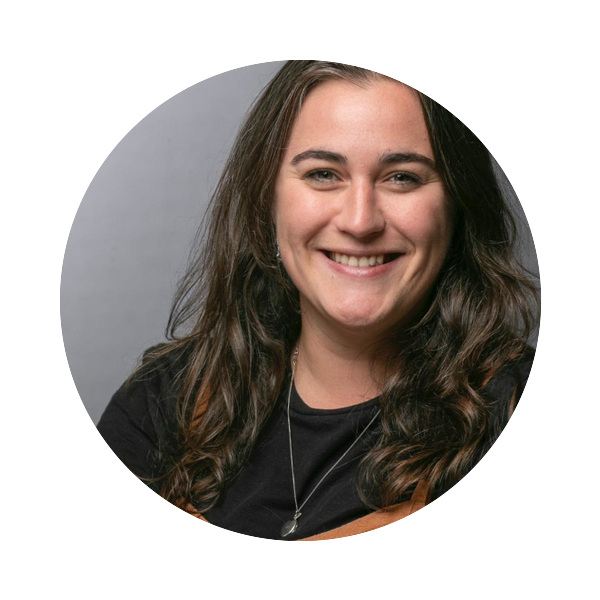
By Dr Rachel Harding and Dr Sarah Hernandez August 12, 2024 Edited by Dr Sarah Hernandez and Dr Rachel Harding
We’re back for Day 3 of the Hereditary Disease Foundation (HDF) conference! First up is a session on RNA dynamics - what’s that?! Read on to find out!
Different HTT forms have different effects
Up first is Gill Bates, who will tell us about her work in understanding how somatic expansion causes disease and investigating ideas targeting different forms of HTT, to help develop therapies for HD.
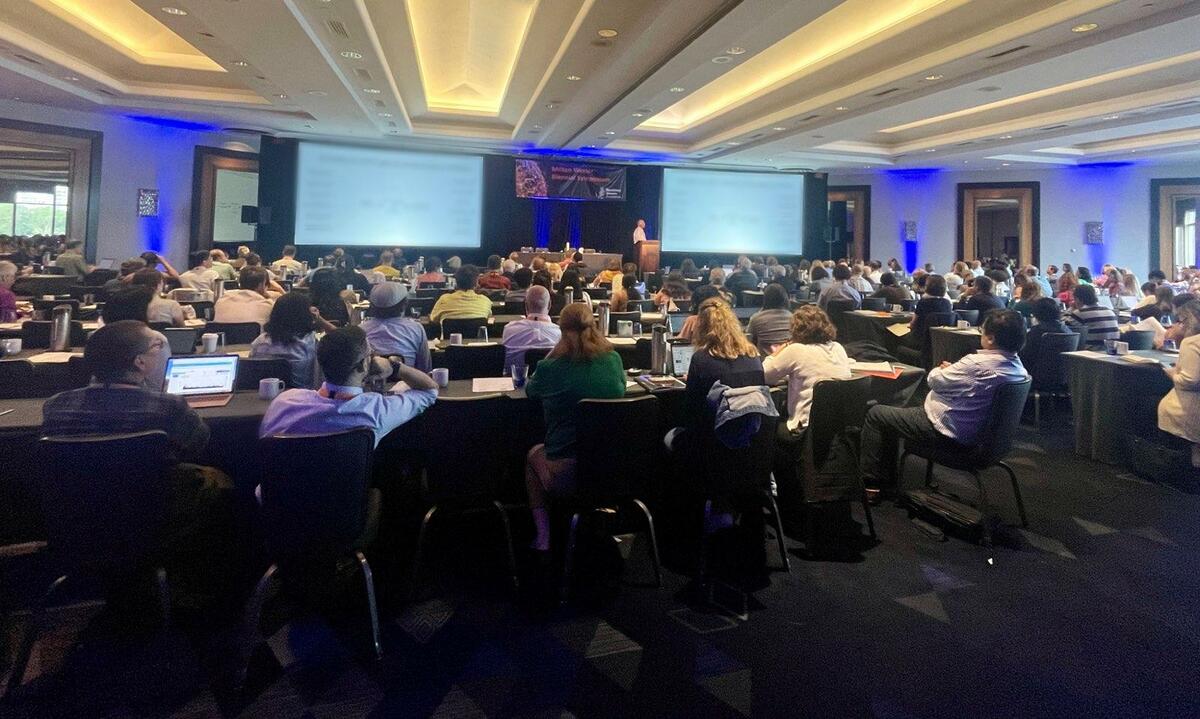
The HTT gene is very long! And sometimes only parts of it get turned into protein, particularly the beginning part. This happens more frequently in HD. It turns out that first little bit - called HTT1a - is quite toxic to cells. Gill’s team research HTT1a in mouse models of HD and they have studied a series of mice with different CAG repeat lengths, spanning mice with a low CAG repeat number to HD mice with very large CAG numbers. Then they measure which forms of the HTT protein are made in these mice.
Interestingly, they find that the longer the CAG repeat is, the more of the HTT1a fragment is produced. So, perhaps, at least some of the disease-associated toxicity is driven by increased expression of HTT1a. They also find more sticky protein clumps of HTT in mice with longer CAG repeats and more HTT1a bits, suggesting HTT1a primarily makes up these protein clumps. Much less of the really big full-size HTT is made in mice with longer CAGs. Together this points to longer CAGs making more of the toxic protein forms (like HTT1a) and less of other forms.
Next, Gill’s team looked at what happened to HTT if they altered the protein with mutations, not in the CAG repeat, but in the protein building blocks directly preceding this region. This changed where and how many protein clumps they could see in mouse models of HD.
So what effect does HTT1a and other HTT fragments have on somatic expansion? This is something Gill and her team are working on in mice. Her team recently published this work. In that work, they used a mouse model with 185 CAG repeats. When they lowered a gene that is linked to somatic expansion, called MSH3, they were able to halt somatic expansion, but the signs and symptoms of HD still developed in the mice.
This might suggest that somatic expansion has to be halted before the CAG repeat reaches these very extreme lengths, like 185. However, those findings are from a specific mouse model of HD. We need more data to understand this better and know if the same thing happens in humans. A limitation of many HD mouse models is that they have extreme CAG numbers from birth so that scientists can see things that mimic symptoms of HD. It’s possible these models mimic juvenile HD, not the more common adult onset form which might be why they don’t see a change in symptoms.
Gill’s team are working with other labs to specifically lower either the full-length version of HTT or the HTT1a fragment, which Gill’s team think might be an ultra toxic form of the protein. Interestingly, they only see an effect if they dose mice when they are young. When they look at the effect of lowering both forms on HTT protein clumping, they find the biggest effect when they specifically target HTT1a. They’re also able to reduce protein clumps more if they treat the mice earlier.
A theme from recent research seems to suggest treating HD early might be our best bet, but that doesn’t mean treatments won’t work for people later on. A great thing about the HD field is that many people are working on a variety of approaches. Our eggs are in many baskets!
Changes to the recipe
Up next is Anukur Jain, who will be telling us about his work on how the RNA message molecule folds within cells and how that process can go wrong.
A brief Bio101 lesson may be helpful here. DNA is made into a message called RNA before it’s turned into protein. Proteins are the functional molecules of a cell, like the product of a recipe. But they can’t get made without the RNA message, it’s the protein recipe molecule.
The RNA messages from genes that have repeat expansions, like the CAG repeat in HD, are prone to folding strangely, causing problems in how the protein is produced from that message. Like if you spill something on your recipe copy and add 1 egg instead of 2. Oops! Ankur is telling the crowd how altered RNA message folding causes the cell to produce different protein forms and fragments. Just like how the product from your recipe would turn out a bit different if the copy you were reading from was altered.
“ A great thing about the HD field is that many people are working on a variety of approaches. Our eggs are in many baskets! ”
Using molecules that are designed to glow under microscopes, Akur can follow these misfolded RNA messages in cells in real time. So over a course of just 10 minutes, he can watch them form structures that look like droplets in the cell. Very cool!
Ankur is interested in understanding if the spelling code of the RNA message affects where these droplet-like structures form in the nucleus of the cell. Using cells making different RNA messages with CAG repeats, his team are trying to find answers to this question.
So far, Ankur has done this work only with messages of pure CAG repeats, not the HTT message itself. This allows him to track how CAG repeats affect droplet-like RNA messages, but it doesn’t answer questions about what’s happening with this process in HD.
Modifiers of expansion and symptom onset
Next up is Darren Monckton, who will be telling us about some cool details about HD genetics. Darren and his team look at the different flavors of the HD gene that people have, and how this affects the path of disease progression they experience.
Darren is able to look in blood samples from people with HD to look at somatic expansions, using very sensitive measurements. In blood, they often only see a single extra CAG, which is very different to the 10s to 100s of extra CAGs we see in some cells in the brain. Looking at thousands of samples from people in the Enroll-HD trial, they have tracked somatic expansion over time. The 2 biggest factors contributing to somatic expansion are age and longer starting CAG lengths.
Interestingly, this is also seen in folks with intermediate CAG numbers, corresponding to 27 to 39 repeats. These people are unlikely to present with clinical HD symptoms in their lifetimes, yet Darren’s team see that they also have somatic instability. Darren thinks that this means that somatic instability per se is not enough to trigger disease. Perhaps we need the more extreme somatic instability that we see in people with adult-onset HD, usually with 40-50 CAGs, to initiate signs and symptoms of disease.
Some people experience more or less somatic expansion than we might expect on average. Using data from 1000s of people with HD, Darren’s team can pull out the genetic modifiers which affect the rate of somatic expansion. In this dataset, we can see many of the same genes previously identified as modifiers of HD symptom onset - many of which are genes critical for repairing DNA, in particular the little loop out structures that scientists think are commonly formed in repeating CAG DNA strands.
This is relevant because it suggests the very genes that control somatic expansion might be the same ones that control age of symptom onset. Something that has piqued the interest of many in the field recently! BUT, not all genes associated with DNA repair come up as modifiers of both age of symptom onset and somatic expansion. Why is that? That’s something Darren is interested in understanding.
Darren postulates that this may be related to the amount of each of the DNA repair proteins that are present. The cell can only use so much of some molecules, so more doesn’t always mean a stronger effect. Like a glass can only hold so much water, pouring into an overflowing cup doesn’t mean you’re adding more water.
Many of the hits are helper proteins which assist in DNA repair and processing, so their role is perhaps more subtle in somatic instability. Clearly this is a complex process, so HD scientists are going to be busy figuring this all out.
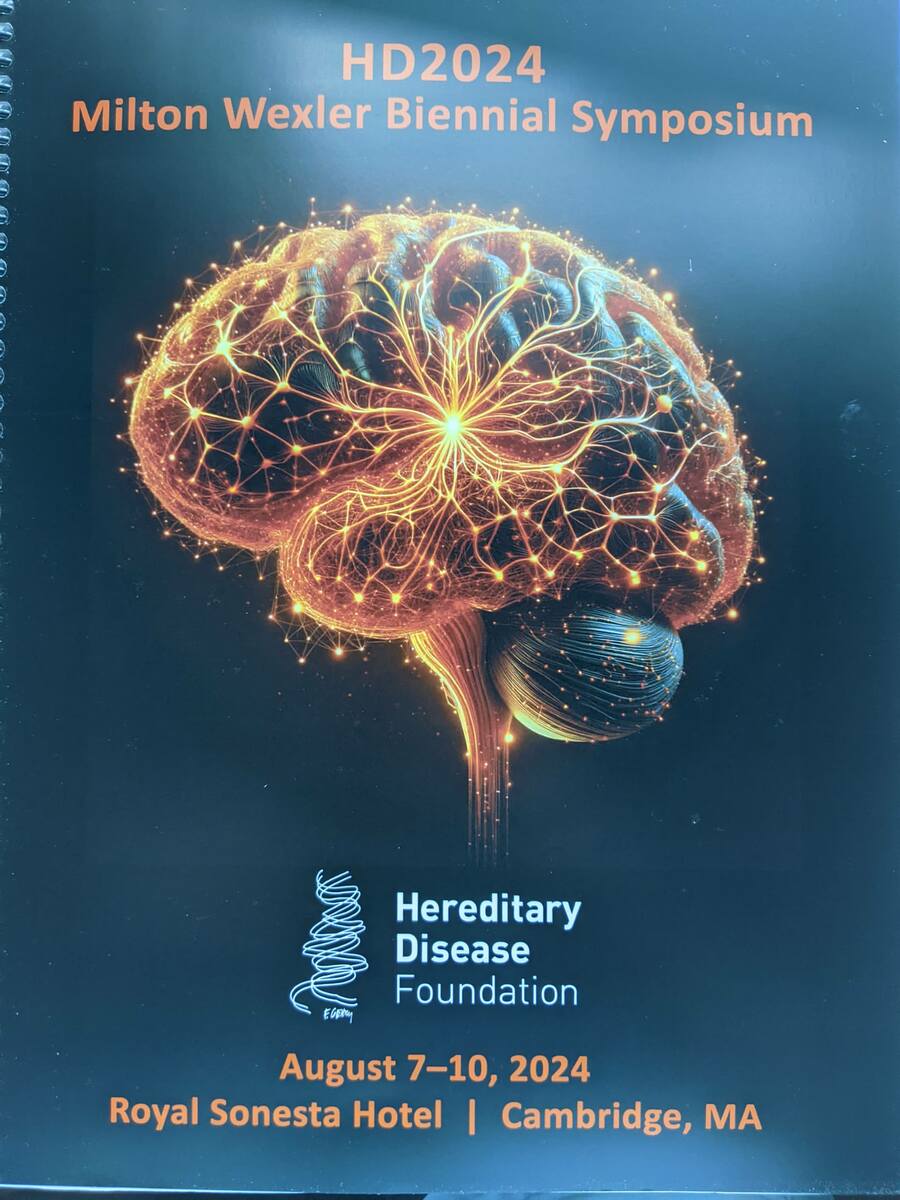
Darren’s also told us that the HTT gene itself modifies somatic instability! This is not caused by the CAG repeat, but by other changes in the HTT gene letter code, and in the DNA regions which surround the HTT gene in the genetic code. Altogether, this suggests that there are likely different mechanisms all working at the same time to alter the rate of somatic expansion and the onset of clinical symptoms. HD is often called the most complicated single gene disease - we believe that is probably true!
Darren’s team also looked at another CAG repeat disease, called SCA3. They see somatic instability in samples from these people too at the affected gene, ATXN3, but the effect is not as strong as it is in people with HD, at the HTT gene. So, like we’ve heard from several other talks, defining these processes in one disease will have implications for other diseases.
Big data to solve big problems
Next up is X. William Yang. His lab creates mouse models of HD that he shares with researchers across the globe to study the disease. In his talk today he is going to be talking to us about why he thinks some cells seem to be sicker than others in HD.
William is showing a super cool movie that moves through a mouse’s brain with markers that glow to show where the sticky HTT protein clumps are. They’re primarily in the center of the brain, in the striatum, and at the outer wrinkly edges, in the cortex. They are looking to see how the clumps match up with where they see somatic expansion in different parts of the mouse brain, and how this impacts which genes get turned on and off. This way they can try to unpick why some cells might get more sick in HD.
William’s team did a massive experiment where they switched off 100+ different genes in their mouse model. Wow! This is a ton of work that will generate lots of useful data for everyone in the field. They focused on switching off genes that they think could be controlling the global changes of genes that get turned on and off in HD.
Once they did this, they looked to see how each gene being switched off affected signs and symptoms of HD. When they switched off many of the DNA repair genes from modifier studies, this made things better - good news for folks working on these as drug targets!
And if that experiment wasn’t big enough, William and his team then looked at those mice using a single cell analysis, looking how each cell in the mouse brains was affected. In line with what others have shown, the striatum was the most affected area of the brain.
William is highlighting data from a specific experiment where they switched off expression of MSH3, a popular target since it influences somatic expansion in HD. When MSH3 was lowered, sticky HTT protein clumps were reduced in his mouse model.
Connecting all of this together to work out exactly what is going on in HD and which events happen in which order is still a tough task for HD scientists to tackle. There is not a clear consensus… yet! But there are lots of smart folks, like those in William’s team, all on the case.
Working together
“And perhaps the underlying theme of the conference so far has been that there are lots of similar mechanisms across diseases that could help inform treatments for many disorders. ”
Up next is Anna Pluciennik, who studies DNA and is looking into a gene called FAN1, which has been shown to modify when HD symptoms might begin. People who have higher amounts of FAN1 get symptoms of HD later than those with lower levels. But why is that and how does that happen? That’s what Anna is interested in finding out!
Anna’s lab doesn’t work with cells in a dish or with mouse models of HD, but only with the precise molecules they are interrogating. In this type of reduced system, they can get into the nitty gritty of exactly how all these molecular machines are working. It turns out that in order for FAN1 to do its job repairing DNA, it needs to work together with other protein molecules. Anna’s team have elegantly defined exactly which proteins are needed for FAN1 to work. We previously wrote about Anna’s work.
Anna’s team used powerful microscopes to look at FAN1 bound onto DNA and one of its partners, called PCNA. By collecting lots of images, they were able to generate a detailed 3D model of the complex of molecules, and so can work out exactly how they work together. She shows that the CAG repeat extrudes out from the DNA helix, and bends awkwardly when it binds proteins like FAN1 and PCNA. This allows FAN1 to precisely chop the DNA nearby the extrusion to begin the DNA repair process and fix the funny looking extrusion.
Anna is teasing out exactly which letter in the genetic code of FAN1 allows it to bind and chop these extrusions - a very high level of detail! She can map some of the variations identified in large genetic studies to her model and test these forms of FAN1 in a test tube. This is cool as her team are able to work out exactly why some genetic variations affect the FAN1 protein, providing great evidence for why these variations impact HD progression.
Learning from others
Our last talk of Day 3 is from Alice Davidson, who studies another repeat expansion disease which affects the eye, called Fuchs dystrophy. This is one of the leading underlying reasons for why some people might need a cornea transplant. Alice and her team have been researching the underlying genetics of this condition to figure out what is going on. In a gene called TCF4, there is a CTG repeat. If this is expanded beyond 50 repeats, then people are at much higher risk of developing Fuchs.
Alice believes that Fuchs could be a good system to test drugs that generally target repeat diseases, given its late onset and the ease of delivering drugs to the eye compared to the brain or muscle for example.
There are a lot of parallels between Fuchs and other repeat diseases, like HD, including toxicities observed with RNA message molecules, sticky protein clumping, and other features, like somatic expansion. Similar to the findings of Darren’s team that we covered earlier, Alice shows us that there is more instability in people who inherit a longer repeat. They next looked to see what might be causing repeat instability.
Alice and her team are interested in defining some of the underpinning mechanisms that lead to Fuchs. And perhaps the underlying theme of the conference so far has been that there are lots of similar mechanisms across diseases that could help inform treatments for many disorders.
That’s all for the research updates for Day 3 - we’ll be back tomorrow for updates from the last day of the conference!