Electron beam snaps best images yet of Huntington's disease protein
Electrons enable huntingtin protein, the cause of Huntington's disease, to be visualised at highest resolution ever
By Tom Peskett June 21, 2016 Edited by Professor Ed Wild Originally published on June 20, 2016
Figuring out the shape of a protein can help scientists understand how it works and what goes wrong in disease. Huntingtin, the protein that causes Huntington’s disease, has been an elusive target. A recent study using electron microscopes offers a striking glimpse of huntingtin, paving the way for future work.
Seeing is believing
Proteins are the machines that perform all the important day-to-day jobs that our cells need to carry out. The precise 3D shape or structure of a protein gives it the ability to perform its specialized job.
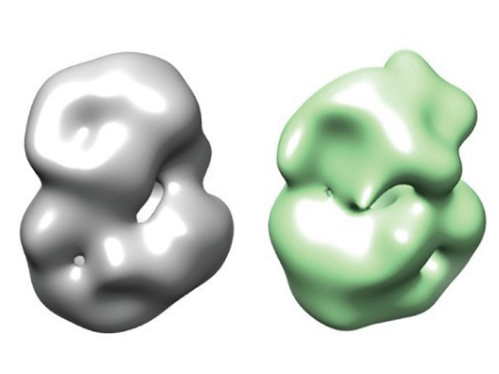
Huntingtin, the protein that causes Huntington’s disease, has a ‘tail’ made up of a chemical called glutamine, one of the protein building blocks. When the tail is excessively long, due to a mutation in the DNA blueprint for huntingtin, it causes HD. We don’t yet understand exactly how the extra glutamines change huntingtin from a normally functioning protein to one that causes harm. So far, scientists have tried to work this out by studying mainly what huntingtin does, but perhaps if we could see huntingtin, this would give us some important clues.
Why do we want to know what huntingtin looks like?
There are two main reasons for trying to figure out what huntingtin looks like.
First, if we knew huntingtin’s structure, it is likely this would give us hints about how normal huntingtin works and how this goes wrong in HD. This information could be used to direct future research and speed up the discovery process.
Second, if we had very detailed information about the structure of huntingtin, it might be possible to design drugs that would target the toxic ‘mutant’ huntingtin.
Why’s it so hard?
Proteins are too small to see using straightforward methods. If you could expand a single molecule of huntingtin protein until it was easily visible, say to the size of a watermelon, that would be like expanding a watermelon until it was as wide as the USA. Even the best light microscopes available are nowhere near powerful enough to see a single huntingtin molecule.
“It seems that huntingtin is flexible - it likes to jiggle around and adopt different shapes ”
That’s where electrons come in. Electrons are almost inconceivably small particles that are found orbiting the edges of atoms - and they can be used in microscopes. While light passes around a single protein, barely noticing it, electrons feel a strong push from the protein, which can ultimately be detected and used to make an image. So an electron microscope was the instrument of choice for an international team of researchers led by Ihn Sik Seong of Harvard Medical School, who wanted to look at huntingtin’s structure.
First, they genetically engineered insect cells to produce human huntingtin. They were then able to extract the huntingtin protein, removing all of the other proteins that it was mixed with in the insect cells. The huntingtin protein was then placed on a small metal grid and put in an electron microscope. They then took pictures of the grid using the electron microscope, which gave them grainy images containing small white objects: their first view of the individual huntingtin molecules.
Huntingtin likes yoga?
So far so good, but the researchers were faced with another problem. If you were to take the pictures of the individual huntingtin molecules and line them up side-by-side, they would all look slightly different. To put it another way, it seems that huntingtin is flexible - it likes to jiggle around and adopt different shapes.
To improve their view of huntingtin, they turned to a technique used by most electron microscopists, called ‘averaging’. They used a computer to combine about 10,000 images of individual huntingtin molecules to bring out the features most common to all. This gave them a much better idea of what an ‘average’ huntingtin looks like. This would be similar to overlaying lots of pictures of peoples’ faces – you would lose the detail of their individual expressions but would keep the really important features such as the fact that each person has two eyes, two ears and a mouth.
Their final 3D structure shows that huntingtin is probably made up of two ‘arms’, which are linked together by a ‘hinge’ that allows the arms to bend towards and touch one another, producing something that overall resembles a sphere. When the researchers compared the structures of normal and mutant huntingtin, they saw a small difference in shape, suggesting that the HD mutation affects huntingtin’s structure in a subtle way.
In a different experiment, the researchers used a chemical to randomly link closely spaced regions of huntingtin to itself. Looking for the chemical links, they could tell which regions of the protein were likely to sit next to one another. They found that when they increased the number of glutamines in huntingtin’s tail, the bending of huntingtin’s two arms changed. The arm with the glutamines curled up less and the other arm curled up more. Exactly what this means is not yet clear, but it does suggest that a small change in one part of the protein can have consequences for the protein as a whole — a potentially important finding and one that may help explain how glutamine-lengthened huntingtin is altered in HD.
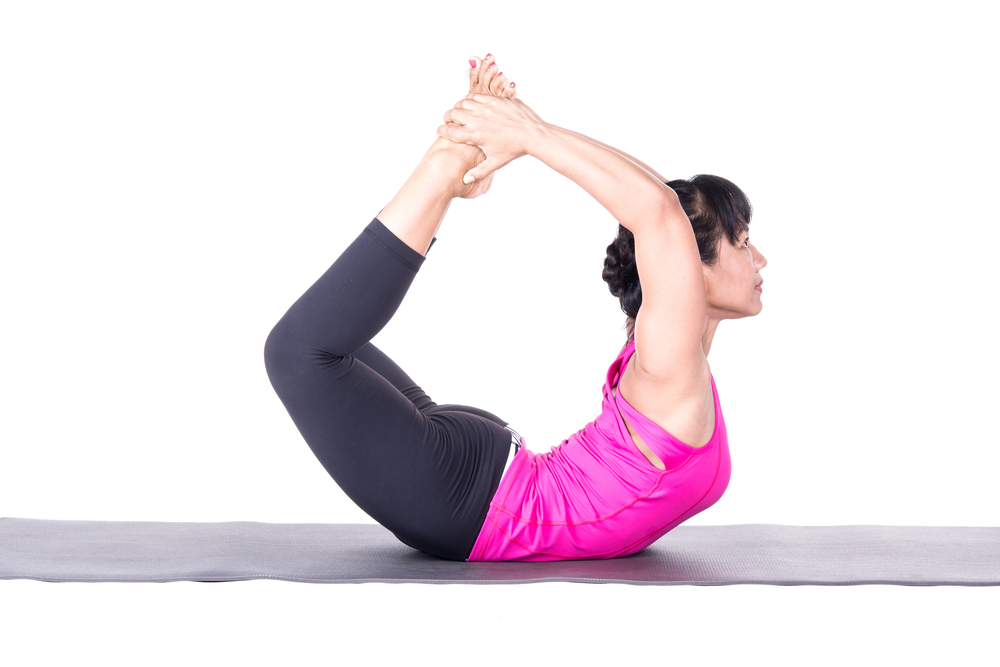
Limitations and next steps
Huntingtin turns out to be very difficult to work with so the researchers had to use a chemical to stabilize it, and another chemical to make it more visible in the microscope. These chemicals could have altered huntingtin’s structure slightly and affected their interpretation of it. One way of overcoming this is to embed proteins in ice, eliminating the need for those other chemicals, and future experiments will likely address these issues.
It’s also worth remembering that the huntingtin in this experiment was purified and put in a test tube, so the range of shapes huntingtin can make is probably even greater in the human brain where it interacts with other proteins.
What’s the next step?
This study provides an exciting glimpse of huntingtin, but there is still a lot of work to do. The structure obtained by Seong and colleagues is certainly not detailed enough to be useful for designing drugs against HD. However, modern electron microscopes are getting better at capturing the structure of proteins, and future studies will aim to do just that with huntingtin. A detailed knowledge of huntingtin’s structure could be crucial in understanding what goes wrong in HD. Now researchers are beginning to pull back the curtain on huntingtin.