Hereditary Disease Foundation (HDF) conference 2024 – Day 2
Read updates from clinical trials and scientific research on Huntington’s disease from Day 2 of the 2024 HDF Milton Wexler Biennial Symposium #HDF2024
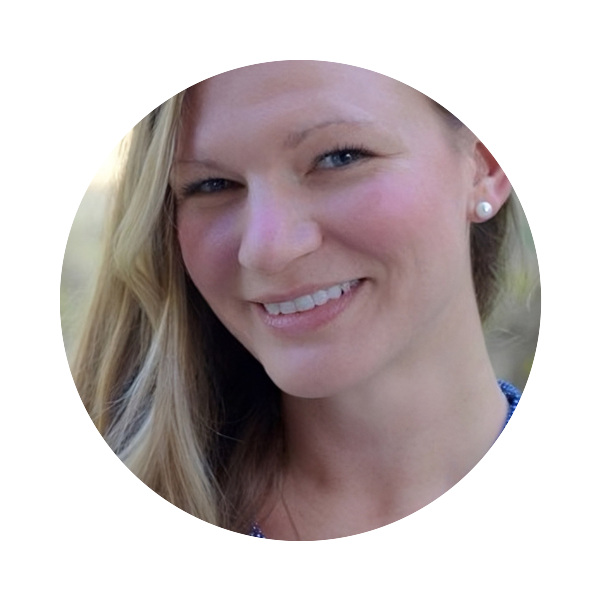
By Dr Tamara Maiuri, Dr Sarah Hernandez, and Dr Rachel Harding August 10, 2024 Edited by Dr Sarah Hernandez and Dr Rachel Harding
Welcome to Day 2 of the Hereditary Disease Foundation (HDF) conference! The morning was spent listening to an interview between a neurologist and their patient living with HD. All HDF meetings begin this way, to better connect scientists with the people who matter most, those living with HD.
Different flavors of HTT
Up first is Tony Reiner, who studies the structure of the brain and how it changes in HD. Interestingly, HD doesn’t affect the whole brain equally. There are certain parts that are more vulnerable - specifically, a region called the striatum, which is found almost exactly in the center of the brain.
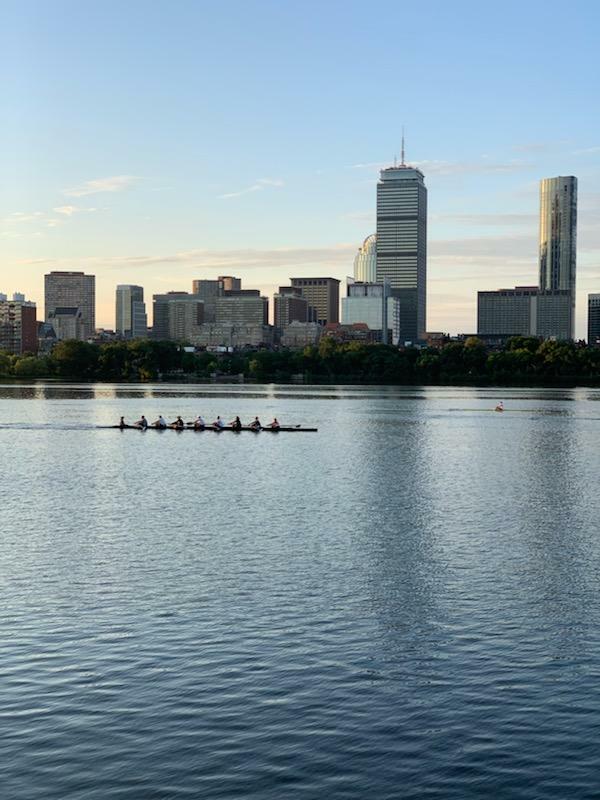
Cells found within the striatum tend to get sick and die in HD, causing this part of the brain to get smaller as the disease progresses. The outer wrinkly bit of the brain, called the cortex, also shrinks in HD.
The gene that causes HD produces a protein (huntingtin, HTT) that is quite sticky, and clumps up in the brain. Tony’s work examines brains generously donated from HD families to track where these sticky clumps are found throughout the brain.
Tony’s lab has studied donated brains to measure the loss of different brain regions at different stages of disease, to ask whether the most vulnerable regions are those with the most HTT protein. Surprisingly, this is not always the case.
In fact, certain cells within the brain that aren’t very vulnerable to HD produce lots more HTT protein than very vulnerable cells in the striatum. Quite surprising!
If not the mere presence of the HTT protein, then what causes cells in the striatum to be so vulnerable? To answer this question, Tony is meticulously tracking different forms of the sticky HTT protein throughout the brain.
Like chocolate can come in different forms (hot chocolate, bar, chips), proteins can come in different forms too. These different protein forms can perform different functions, good or bad, perhaps making some forms of the sticky HTT protein toxic.
Knowing which form of the protein is found in which areas of the brain will help researchers understand if certain types of HTT are more toxic than others, which could help with understanding the details of how HD affects the brain.
It’s not all about neurons
Up next is Osama Al Dalahmah, who is another brain pathologist - someone who studies the structure and function of the brain. He’ll be talking to us about his research on a star-shaped cell in the brain called astrocytes.
Neurons get a lot of attention in HD - and rightfully so! Neurons are the cell type that send electrical signals to help us think, move, and feel. And they’re the cell type most affected by HD. But neurons aren’t the only cells that make up the brain.
Astrocytes connect to neurons to help maintain the environment of the brain to keep neurons happy and healthy. We’ve previously written about astrocytes and the role they play in HD.
Osama’s group is asking how astrocytes in people with HD may be different and if astrocytes may even be protective against the disease! Using donated brain samples and cutting-edge technology, they can study minute differences in each astrocyte cell within a brain sample.
In particular, they are looking to see which genes get turned on/off in the astrocytes found in the brains of people with HD. There seem to be some patterns that make up a “molecular signature” for astrocytes in HD. Interestingly, it seems that cells with some of these molecular signatures are actually adapted to work to help protect the brain during HD.
Osama likens neurons to crowd surfers being carried by the crowd, in this case, supporting astrocytes. Some astrocytes in people with HD support crowd surfing neurons, but others, without the right signature, allow for stage dive fails. No fun for crowd surfing neurons!
“As we’ve heard already at this meeting, a rising tide lifts all ships - finding treatments for one brain disease could have implications for others, including HD. ”
The cellular trash bin
Up next is Joan Steffan who is going to be talking to us about her research looking at what the HD protein does normally. We know the HD protein, HTT, doesn’t work well in disease. But the HTT protein has lots of important jobs to do in healthy cells and Joan, and the other speakers of this session, are interested in investigating these functions.
Joan is studying the role of the HTT protein in cleaning up components of the cell that are no longer needed. This process, called autophagy, is very important to keeping cells healthy. Joan found that HTT works with lots of protein friends in the cell to take out the cell’s trash.
Many proteins involved in autophagy bind to the cell trash via a molecular tag. So Joan’s team asked if the HTT protein could also bind this tag. Turns out it can in a test tube!
The HTT protein is huge, one of the largest that our bodies make. Joan and her team have mapped the exact part of HTT which binds onto this tag. The tag-binding region is right on the edge of the donut structure of this massive molecule.
Looking closer, Joan asked what cellular trash might be bound by the HTT protein. She found that lots of these were proteins whose job is normally to bind genetic message molecules, called RNA. Joan has lots of ideas about what this might mean for HD biology.
She also found that the expanded form of HTT, which causes HD, interacted more tightly with the trash tag. This gives us more clues about the normal role of HTT and what might be going wrong in HD.
Huntingtin’s BFF - HAP40
Next up is HDBuzz’s very own Rachel Harding! She’ll be telling us about cool new tools she’s using to better understand the structure of our favorite protein.
Rachel reminds us of how very big the HTT protein is. She’s very interested in its shape: one half looks like a donut, which is connected to the other half through a bridge. These two halves are held together by another protein called HAP40.
Rachel’s lab is very good at producing the HTT protein in a test tube. This is used by labs all over the world to understand what the HTT protein does.
An important part of understanding what a protein does is knowing what other proteins it interacts with. One of the tools used to discover these interactions is antibodies. So it’s very important that the HTT antibodies are of good quality. The good news is, we have some great antibodies. The bad news is, some of the antibodies regularly used by HD research labs are not so great.
To make sure we’re using the best tools possible, Rachel is developing an alternative to antibodies called macrocycles. These are small molecules that bind to HTT very tightly and can be attached to other things like fluorescent tags that will make the HTT protein glow. Very cool!
Using several fancy technologies, Rachel’s group is figuring out exactly where each macrocycle is binding on the HTT-HAP40 structure.
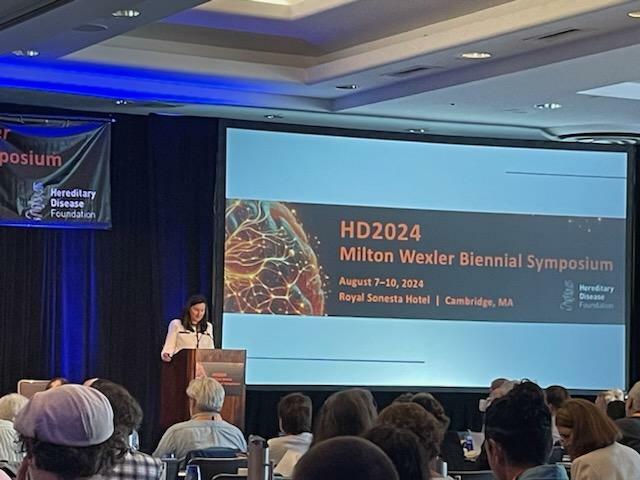
Macrocycles can be used for much more than just studying HTT in a test tube. They can also track HTT in cells, which will be pivotal in helping researchers understand the function of HTT and what might be going wrong in HD.
They may also be used to find “pockets” in the HTT protein that would make good drug targets.
Picking up speed
Next up is Bob Handsaker who will talk to us about somatic expansion in HD - the idea that in some cells, the CAG repeat can get longer over time. HD scientists are trying to figure out how this might contribute to the path of disease progression, a very exciting area of research.
Bob and the team he works with at Harvard have built a model of how they think somatic expansion happens in cells, first in a slow and then in a rapid phase. They have collected evidence from brain tissues analyzed from people who have passed from HD that they believe supports this model.
Next, Bob tells us about changes to genes being switched on and off and how this correlates with somatic expansion of the CAG tract. Interestingly, they don’t see much difference until the expansion becomes very large, around 150 CAGs.
After the cells reach this very long CAG repeat length (which takes decades), they start to see accelerated changes in genes that are turned on and off, leading to toxicity in the cell, and eventually death of those cells.
The model Bob is proposing is somewhat in contrast to data published by other scientists, many of whom are in the room - but this is what conferences are for, to discuss these hot topics and see how the collective evidence shakes out, to move the science forward.
Interestingly, when they dig into the data to see which genes are turned off in neurons from the striatum (the very center of the brain) they’re genes associated with “cell identity”. This means the cells, in a way, lose their ability to tell what kind of cell they are.
Bob and the team also looked at the protein clumps that they see in the brain and how these change over time. Their modelling and analysis suggest that this is a late feature of HD, happening in just a subset of cells in the brain.
Overall, the model Bob proposes suggests why HD might take decades to develop and they hope it can be used to develop better therapeutics for HD, or to track how new drugs might slow or halt HD.
Different disease, similar effects
Up next is Harry Orr, who works on a different CAG repeat disease called spinocerebellar ataxia 1 (SCA1). While there are similarities with HD, there are also differences. SCA1 typically has adult onset, causes movement changes, and problems with thinking. Also like HD, there is no treatment.
One major difference is the primary cell type affected. While HD primarily affects neurons of the striatum, SCA1 primarily affects a different type of brain cell called a Purkinje cell in an area of the brain called the cerebellum.
“There’s very cool science being done in the HD space! ”
Harry’s lab has been working on developing mouse models of SCA1 to better understand this disease. They are using these models to look at somatic expansion in different parts of the brain
It seems ongoing CAG expansion isn’t unique to HD, but may be a common feature in several diseases, including SCA1. As we’ve heard already at this meeting, a rising tide lifts all ships - finding treatments for one brain disease could have implications for others, including HD.
Location, location, location
Our last talk of day 2 is from Longzhi Tan whose talk is titled, “3D genome architecture across the lifespan and in HD” - sounds like it will be high tech!
Each of our cells carries the entire genome – all of our DNA – in its nucleus. Tan analyses the shape of this DNA at the single cell level. Measuring the shape of DNA is incredibly difficult. This is because DNA shape differs cell to cell, so shapes within 2 cells aren’t the same.
Tan developed his own technique to solve these challenges and define the shape of DNA across many cells. Combining computers with microscopes gives a high-tech solution, allowing him to back calculate DNA shapes within the nucleus.
He’s showing the crowd super cool videos that have caused audible murmuring throughout the audience. There’s very cool science being done in the HD space!
Each cell uses different genes to do its job. Tan is explaining how the genes used by one cell type move toward each other in the nucleus. For HD we’re of course interested in brain cells. Tan can tell the difference between diverse brain cell types simply by looking at the position of their genes. Wow!
Tan is using his cool technology to study HD by asking if the disease affects the position of DNA within a cell and if that may alter which genes are on or off. He is currently working on these questions in mice that model HD. They have found that the biggest differences in the DNA’s 3D shape happen in the very cells that are vulnerable in HD!
Tan is also looking at how DNA shape changes when he turns off a gene associated with somatic instability, called MSH3. Turning off MSH3 rearranges the DNA location so that it more closely matches cells without HD.
Overall, Tan’s work is a super cool debut of new technology that can be used to analyze HD in very fine detail.
That’s all from us for day 2 of the conference! We’ll be back for day 3 to share updates about cells other than neurons, somatic instability, and DNA repair. Stay tuned!