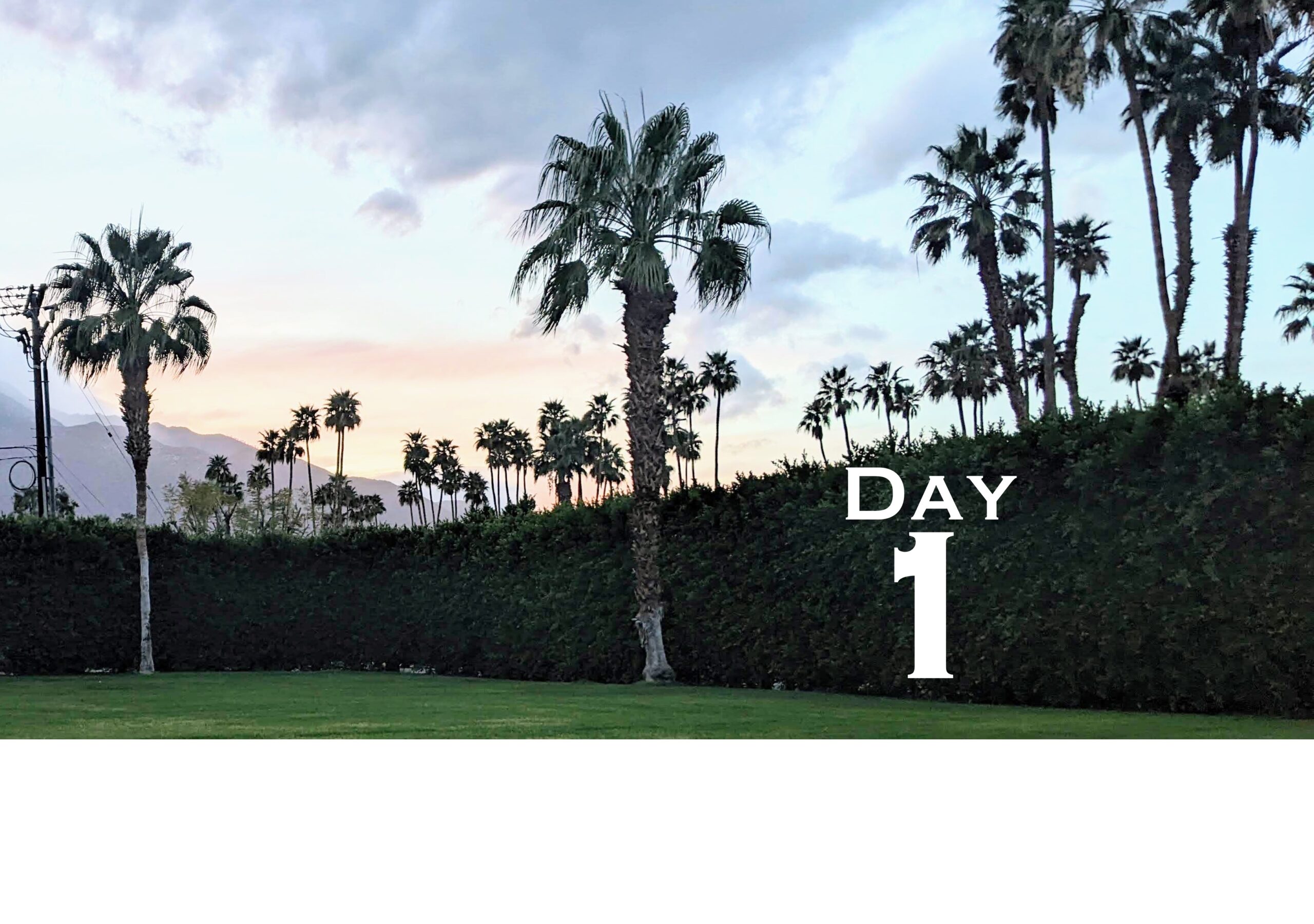
Huntington's Disease Therapeutics Conference 2024 – Day 1
Check out research updates from Day 1 of the 2024 HD Therapeutics Conference #HDTC2024



The HDBuzz team recently convened in Palm Springs, California, along with hundreds of other scientists from all over the globe, for the 19th Annual HD Therapeutics Conference, hosted by the HD research foundation CHDI. From Tuesday February 27th through Thursday February 29th, we live-tweeted dozens of scientific talks by world experts in Huntington’s disease research, from bench scientists to clinicians. We’ve compiled our tweets into a summary of the entire conference, beginning with Day 1.
Huntingtin Homework: Teaching an Old Dogma New Tricks
The first session of the conference focused on huntingtin, the gene that expands to cause HD. The meeting kicked off with Gill Bates, who reviewed what we know about the genetics of HD, which is that the length of CAG repeats in the huntingtin gene dictates whether people will develop HD. More recently, lengthening of the CAG repeat in some brain cells, known as somatic expansion, has been implicated in driving the disease.
Image credit: Ed Wild
There are many unanswered questions about exactly how the CAG expansion results in the death of brain cells. This session addressed a wide range of approaches, at the epigenetic, RNA, and protein levels.
Karine Merienne: DNA tags in the striatum
The first speaker of the conference was Karine Merienne from the University of Strasbourg who told us about changes in certain types of markers in HD associated with aging. Karine reminded us that it is still a mystery why the nerve cells of the striatum are so vulnerable to the CAG expansion in the huntingtin gene. One hypothesis she is investigating are changes in how genes are turned on and off.
Karine’s research looks into how so-called epigenetic changes occur in HD. These aren’t changes to the DNA code itself but certain markers which affect how DNA is packaged. This in turn changes which genes are switched on or off, which affects the properties of cells, making a nerve cell a nerve cell, or a skin cell a skin cell.
The epigenetic markers change with age in all people, not just folks with HD. Karine’s team found that certain epigenetic markers are altered further in mouse models of HD. Using cutting-edge technologies, they were able to look at changes to markers at the level of single brain cells from a mouse. They saw that nerve cells from the striatum, the vulnerable brain region in HD, were missing an important epigenetic mark.
This gives us insight into why cells in the striatum might be more likely to get sick in people with HD. Perhaps if we can fix these epigenetic marks, we could make the cells better again? We will see; there are a lot of smart folks working on this!
Yinsheng Wang: chemical tags wreaking havoc
The next talk of the morning came from Yinsheng Wang, who is based at the University of California, Riverside. Yinsheng investigates how genetic message molecules are modified with chemical tags in HD and other CAG diseases.
The repeating CAGs in the message of the huntingtin gene can be “tagged” with extra molecules. In the course of HD, the extra tags can inappropriately recruit proteins in the cell, sopping them up and keeping them from their normal jobs. Interestingly, these changes to the tags on the huntingtin message molecule seem to happen most often in the striatum in HD mice. Could this be another reason why these brain cells get the most sick?
Special molecular machines add or remove these tags from the huntingtin message molecule. Yinsheng and colleagues figured out which of these machines was responsible for the changes they could measure. If they got rid of the machine, the message molecule tags went back to normal.
They also found that changes in molecular tags alter the ability of the huntingtin message to stick to a protein called TDP-43, which plays a role in other neurodegenerative diseases. (Like huntingtin, TDP-43 can also form clumps.) Huntingtin along with its CAG repeats and tags can affect formation of these clumps. This team’s data suggest that expanded repeats on the huntingtin message molecule led to clumping of TDP-43.
Yinsheng explains yet another way that chemical tags on the huntingtin message molecule may wreak havoc in the cell: by causing the message molecule to get off kilter and make toxic proteins it is not meant to encode. Maybe if we can restore the way the HD message molecule is tagged by these molecular machines, brain cells will get less sick. This is an interesting new avenue for drug discovery research in the HD field.
Jeff Carroll: how low should we go?
We also heard from HDBuzz’s very own Jeff Carroll. Jeff’s lab, which is based at University of Washington, has been busy investigating HTT-lowering in mouse models of HD. Jeff’s lab is asking what they can do to help speed up and improve upon clinical huntingtin lowering strategies. One major question is how much to reduce huntingtin and in which types of cells to improve symptoms of HD without negative effects.
Key questions Jeff’s team is trying to answer are how low is too low for huntingtin lowering, and how low do we have to go to have a positive effect. Experiments in mice have shown that going too low might not be very good. One of the projects Jeff’s lab has been working on is looking at huntingtin lowering in adult mice. This represents what we are doing in the clinic, giving adult people with HD different types of drugs to lower huntingtin protein levels.
Jeff’s lab showed that it was not safe to completely remove huntingtin in the brains of mice – they literally had holes in their brains! Of course, complete removal of huntingtin in human brains is not what clinical trials are aiming for. The trick is probably going to be getting the balance right. Jeff reiterates this does not mean that huntingtin lowering is a bad idea per se, we just need to figure out how low to go.
We know from genetic studies that 50% (what most clinical trials are aiming for) seems to be ok in people and animal models of HD, but perhaps below that is not a good idea. One interesting thing Jeff’s group noticed was that lowering huntingtin with ASOs in mice seems to alter somatic instability, the expansion of the CAG repeat in some cells. This seems to happen in the liver at very high ASO doses. Figuring out why this is the case has been complicated!
More importantly, would the clinical approaches, which use lower amounts of ASOs, do the same? It doesn’t appear so. But it’s probably something scientists should keep an eye on moving forward.
Next Jeff’s team looked at selective lowering approaches – therapies which only target the toxic huntingtin and leave the regular huntingtin levels untouched. Again they saw that somatic instability seemed to be blocked by these treatments – this could be a cool two for the price of one therapy!
“Discoveries in the realm of somatic instability would have been impossible without the dedication of HD families and their willingness to participate in research.”
Altogether, Jeff’s work is helping us better understand what huntingtin-lowering means at a molecular level, which will help us better understand what’s happening in the current clinical trials, and any extra considerations we might need to think about to give this approach its best shot.
Ileana Cristea: huntingtin and its dance partners
We’re back and up next is Ileana Cristea who leads a research group at Princeton University. They study the huntingtin protein and the other proteins it likes to hang out with in cells, and how these relationships change in HD. This work lies in the area of “omics” research where researchers look at changes in proteins and how they interact across many cells, tissues, and brain areas. There’s tremendous collaborative effort!
Ileana’s talk continues the theme of figuring out why some cells get sick in HD, while others seem to be ok. Her lab looks at the locations of different proteins and which ones hang out together. They are trying to address what drives disease, and what are the consequences of lowering huntingtin levels with drugs. They are doing so by studying what proteins interact in the presence and absence of huntingtin.
They found that in cells from mice which don’t have huntingtin protein, the levels of all sorts of proteins were changed, as well as which proteins were hanging out together. The biggest changes occurred in proteins with jobs in energy generation and DNA repair. The next step was to understand how these changes lead to disease, so they zeroed in on the striatum of HD mice, since this is the region of the brain most affected in HD.
They narrowed down a list of proteins involved in specific cell functions that may give us clues to what goes wrong in HD. After getting a bird’s eye view of networks that interact, they confirmed some of their “hits” in collaboration with scientists who work on other models and techniques, like cells and fruit flies. In different types of cells, different protein buddies of huntingtin are altered, which once again could explain why different cells are affected in different ways in HD.
Not only can Ileana’s team work out which proteins huntingtin hangs out with, but through which surfaces they might all stick together, giving a very detailed molecular view of what is going on in HD. Although this type of study can feel a bit granular, it is actually very important to help us understand exactly what goes wrong in HD. It also helps us identify new genes and proteins which might be good targets for future drug discovery efforts.
Tony Reiner: patterns of huntingtin clumps
The final talk of the morning came from Tony Reiner, who is based at the University of Tennessee Health Science Center. His team have been looking at where the disease form of the huntingtin protein is found in HD human and mouse brains.
Tony first reminded us about the specific parts of the brain which are most vulnerable in HD. The striatum, which is at the very center of the brain, is one of the most affected brain regions, but nerve cells in the cortex and thalamus are also affected. The regions of the brain that make a lot of huntingtin protein are not necessarily those most affected in HD. Regular huntingtin is really found everywhere, so this does not seem to explain why cells in the striatum are so vulnerable.
Huntingtin proteins come in lots of different flavours – expanded, fragmented, clumped, and others. Tony’s group uses antibodies as tools to visualize the different forms of the huntingtin protein in the brain. They started by looking in HD mouse brains, and tried to match that up with tissue from people with HD who generously donated their brains to research after passing away. This selfless act has been critical for generating data that lets us know what’s going on with HD in human brains.
If you’re interested, you can learn more about brain donations from the Brain Donor Project (https://braindonorproject.org/), HDSA, HSC, HD organizations in your home country, and through local academic institutions.
We can measure lots of changes in brain cells over time but which changes are actually causing cells to get sick is still not very clear. Tony’s work shows that increased protein clumps and other measurements don’t always seem to match up with the cells we know get sick. Work from Tony’s lab and others really get into these measurements at the level of different cell types that might one day help us better understand cause and effect.
Targeting the DNA Repair Machinery to Modulate Somatic Instability
Session 2 of the conference focused on ways to combat the expansion of CAG repeats. This happens in some cells as HD symptoms worsen. Lots of new evidence points to the lengthening of CAG repeats as an important driver of cell loss in the striatum, pushing symptom onset earlier. Using blood donated by thousands of individuals with HD, scientists have been able to perform large genetic studies (known as GWAS) to determine other genes that affect HD onset. Notably, many of these genes are involved in something called DNA repair.
DNA repair is a process where little molecular machines repair mistakes or changes in the spelling code of DNA. One of those changes that happens in HD is the increase of the CAG repeat. Some people have DNA repair machines that are great at catching mistakes, while others have machines that aren’t so good. When the DNA repair machinery fails, the CAG repeat can lengthen in some cells over time.
Many HD scientists now believe that by targeting DNA repair genes, it could be possible to slow down the lengthening of CAG repeats in vulnerable brain cells, ultimately delaying onset or slowing the worsening of HD symptoms. More and more scientists and companies are now seeking, confirming, and testing different players within the machinery that repairs DNA, to determine what goes wrong when CAG repeats get longer, and try to prevent it.
We’ll be throwing around lots of acronyms and names of proteins known to be involved in the DNA repair process. These proteins work together to repair DNA in different ways and may go awry in HD. Scientists are developing genetic drugs to increase or decrease their levels and stop CAG expansion.
Maren Thomsen: the shape of DNA repair machines
The afternoon’s first speaker was a structural biologist, Maren Thomsen from a company called Proteros Biostructures. She is studying the shape of a protein called MutSꞵ which is involved in DNA repair. Using special microscopes they can see exactly how the protein is organised in 3D space which can help scientists figure out how it works.
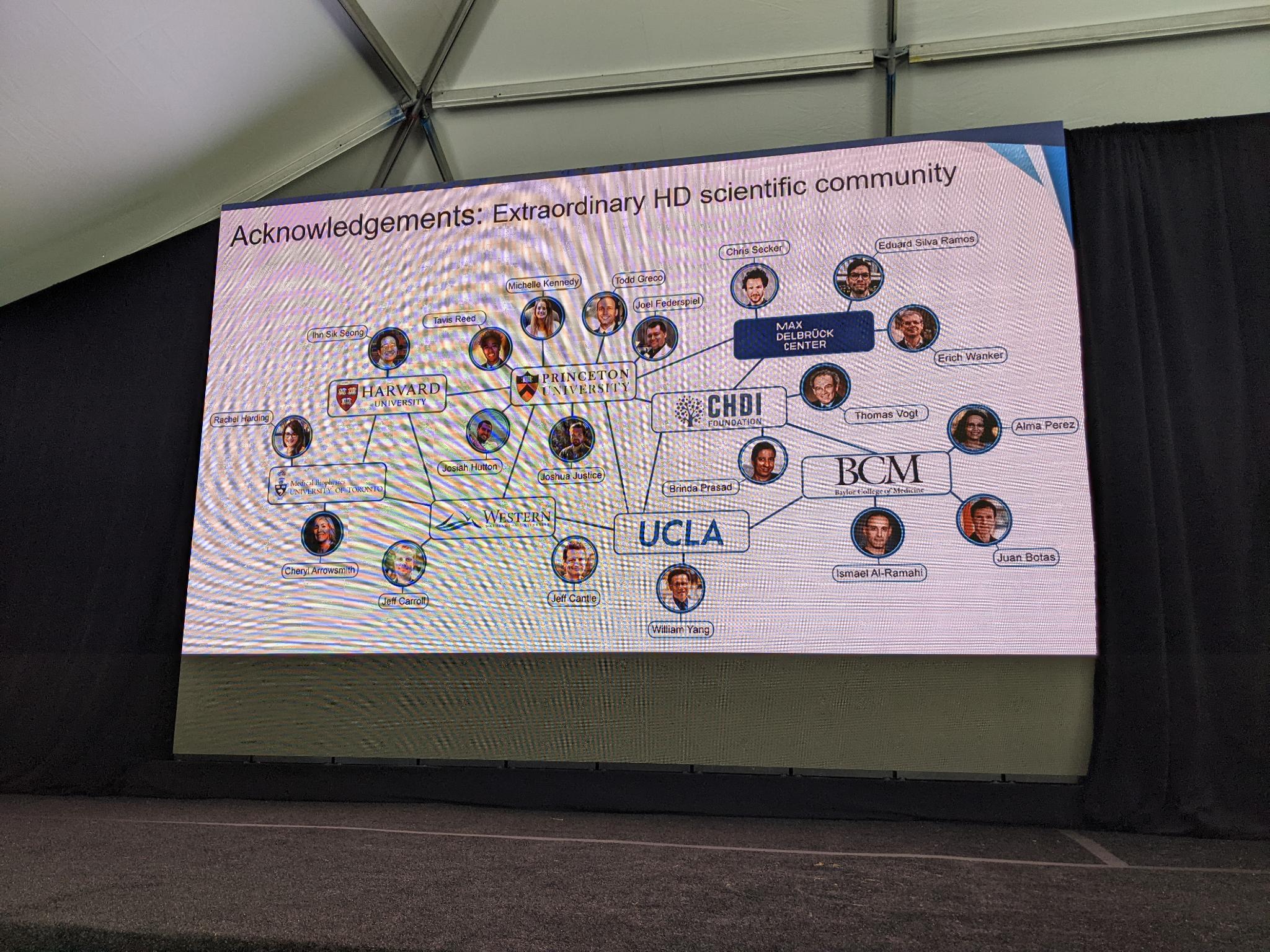
Image credit: Ed Wild
Ideally we would like to stop the activity of MutSꞵ, and there are different ways to do this. Maren uses the analogy of trying to stop the movement of a bicycle: you could stop the pedals, or put a barricade in front of it, or block the wheel from turning. Knowing the details of the MutSꞵ structure allows them to come up with different ways to stop its activity.
Cool microscopes allow Maren and her team to figure out precisely where each atom of the protein is, and how this changes as MutSꞵ goes about its job sliding along and repairing DNA. With this high resolution of information, they can create a super detailed model of how this molecular machine works. It can really move, gripping and opening around DNA like a clamp! When Maren’s team makes changes to the MutSꞵ machine, this changes its grip and motion, and they are learning more about which parts of the protein stick to other players in the DNA repair process.
Maren is also investigating a protein called FAN1, along with its “dance partners.” FAN1 is also involved in DNA repair, and her group has been able to visualize it in new ways with the goal of harnessing it for drug development. With these detailed insights into how all these molecules work together, we can begin to understand why certain mutations found in GWAS might delay or hasten the age of onset of symptoms in HD.
Structural biology techniques illuminate the shape and movement of critical molecules so that we can better understand where and how to intervene in the faulty biology of Huntington’s disease and design drugs that precisely target proteins like MutSꞵ.
Wei Yang: MutSꞵ and long CAG repeats
We heard next from another structural biologist, Wei Yang, who is based at the National Institutes of Health, and is also studying MutSꞵ. The MutSꞵ molecular machine acts on all kinds of repeating DNA sequences, not just CAG repeats. Wei’s team worked to figure out exactly how MutSꞵ binds to these repeating DNA letters using structural biology approaches.
An interesting conundrum is why there is a threshold of CAGs at which the repeats continue to expand over time. Understanding this requires neat experiments looking at CAG repeating DNA and how it binds onto MutSꞵ. Wei and her team found that MutSꞵ much prefers binding onto longer CAG repeats than shorter ones. In fact, if the CAG stretch is long enough, more than one MutSꞵ molecule will bind onto the CAG DNA.
When they look at the CAG DNA stuck to MutSꞵ, they see that MutSꞵ contorts the CAG repeating DNA to look like non-repeating DNA. It works like a vice to bend the DNA into this different shape. Unsurprisingly, this requires a lot of energy. But what does this all mean for HD? Well, when the CAGs repeat enough, like they do in folks with HD, more and more MutSꞵ molecules bind on. Wei thinks this may unintentionally encourage CAG expansion.
Structural biology studies help us confirm findings from people that have revealed the importance of DNA repair in HD. We are learning more about the genes and machinery involved in somatic instability and how it might be linked to symptom onset. Armed with this kind of knowledge, drug hunters can work to find ways to stop MutSꞵ from making mistakes that lengthen CAGs, with the goal of slowing or stopping HD.
In fact, Wei and her team have identified a compound which changes how MutSꞵ sticks onto CAG DNA. Although this molecule is not likely to be a drug, it could be a helpful starting point for drug hunters.
Sarah Tabrizi: exploring DNA repair in human cells
Next up was the legendary Sarah Tabrizi, a physician and scientist from University College London who is involved in lots of basic and clinical HD research. Sarah is using human cells grown in a dish to further test many of the genes identified as important for DNA repair and CAG repeat expansion. There are lots of DNA repair proteins which look like good targets for therapies.
Sarah and her team are using genetic techniques like CRISPR to lower the levels of DNA repair proteins that go awry in HD. Then they see how this affects the levels of other genes, to tease out relationships among the DNA repair machinery. After changing the levels of different DNA repair proteins, Sarah’s team then looked to see how this affected CAG repeat expansion. They targeted genes called MSH2 and MSH3. Lowering these (and others) reduced the level of somatic expansion.
In stem cells coaxed to become brain cells, lowering of one specific DNA repair gene called MLH1 reduced somatic expansion by 78% – wow! She’s also exploring other targets, including a gene called MSH3, which she calls “an ideal therapeutic target.” Her work highlights two repair proteins called MLH1 and PMS1 as potentially interesting drug targets. Working on targets beyond the MutSꞵ complex could be really handy to increase our odds of finding drugs that work.
Nonetheless, Sarah reiterates that MSH3 is her favourite drug target, as knocking it out seems to slow CAG repeat expansion. Furthermore, depleting this protein does not seem to have too many bad side effects, at least in models. Sarah’s lab uses ASOs to lower levels of MSH3, the same type of drug that some companies (like Roche) are using to lower huntingtin. When they lowered MSH3 levels, this slowed down somatic expansion. When they completely removed MSH3, CAG repeats got smaller. This did not affect other DNA repair proteins, which is positive news for MSH3 drug discovery.
Nearly all of this work was done in nerve cells in a dish, which had been created from a blood sample generously donated by a young patient that Sarah was treating before they passed. These discoveries and many others in the realm of somatic instability would have been impossible without the dedication of HD families and their willingness to participate in research. Sarah’s team are continuing this work in a mouse model to see how their MSH3 ASOs fare in a more complex system. Watch this space!
Ricardo Mouro Pinto: exploring DNA repair genes as potential targets
Ricardo Mouro Pinto is based at Massachusetts General Hospital & Harvard Medical School. His team also studies somatic expansion. Patients with increased somatic instability start to have HD symptoms earlier. The genes that influence the onset of symptoms are known as genetic modifiers. Ricardo and others are testing the theory that changing the levels of these modifiers can delay or stop the onset of HD.
Ricardo is using CRISPR to change levels of these modifiers in mice that model HD. He’s screened 60 different genes in mice – that’s a lot of work! What all that work found is that DNA repair genes have a large effect on the length of CAG repeats. This confirms what many others in the field have found. Anytime results are replicated in various labs across the world it strengthens the field’s confidence in that data.
Image credit: Ed Wild
He then moved to human cells grown in a dish to confirm their own and others’ data. They found that lowering MSH3 slows the rate of expansion and lowering FAN1 increases it. They also found that reducing levels of MLH3 and PMS1 halt expansions. Ricardo is also working to check these modifier targets in other diseases. HD isn’t the only disease affected by somatic expansion. So he’s screening the targets he identified in HD in mice that model other diseases, like Frederich’s Ataxia.
Targeting the same protein that is involved in multiple diseases not only benefits more people, but could speed up trial enrollment and drug development. But before we get ahead of ourselves, these targets have to be tested more to ensure they’re having the effect we want! One point researchers have to be aware of is that DNA repair genes are also involved in cancer. Ricardo is being mindful of this and choosing potential drug targets with the lowest chances of leading to cancer in people.
One target Ricardo’s team is particularly interested in is called MLH3. They did some detailed studies to see exactly what part of the MLH3 gene is responsible for somatic expansion in HD. Knowing exactly what part of the gene is responsible for influencing CAG length lets researchers know which spot they would need to target if they designed a drug against MLH3.
Using cool genetic techniques, Ricardo is able to make cells that produce a version of MLH3 that excludes the part of the code that leads to CAG repeat expansion. When he did this, he was able to suppress CAG expansions. He’s now working to use CRISPR to change a single letter in the sequence of MLH3. This is a precise approach that will stop MLH3 from increasing the CAG number while leaving the rest of the MLH3’s functions alone.
This is important because proteins in cells have lots of different functions. Targeting a single function reduces the chances of having unintended consequences. Something that’s critical for drug design! Ricardo’s next steps are to use his new CRISPR approaches to alter MLH3 in mice and human cells in a dish. For this, Ricardo was recently awarded a $1,000,000 grant from the Hereditary Disease Foundation!
Karen Usdin: somatic expansion in other diseases
Karen Usdin from the National Institutes of Health spoke about her team’s work looking at somatic expansion in different types of diseases caused by DNA repeats, beyond HD. There are many diseases caused by repetitive sequences of DNA. Karen’s team primarily focuses on one of those diseases called Fragile X, which is caused by a repeating CGG. However, she’s now also beginning to focus on HD. Woohoo!
Fragile X also has somatic expansion controlled by DNA repair genes. Karen has used mice that model Fragile X to identify which DNA repair genes are important for this process in Fragile X. Karen has focused on parts of the DNA repair machinery called MutL complexes.
Her lab eliminated a piece of the MutL complex, called PMS2. Lowering PMS2 levels stopped repeat expansions in human cells and mice with other genetic diseases. She found similar results with another part of the MutL complex called PMS1. Lowering levels of PMS1 reduced expansions in Fragile X. Combined with data from other diseases, this suggests that PMS1 plays a role in expansion across repeat diseases.
Now Karen is getting to the good stuff – her work in HD! She made a mouse model that had genes for both HD and Fragile X. With this mouse, she was able to look at repeat expansion in both genes at the same time. She wanted to know if expansion of one gene affected expansion of the other gene. Put simply – no. Expansions of the HD gene didn’t influence the gene for Fragile X and vice versa.
She suggests that expansions depend on the balance between different types of DNA repair machinery in different cells. Some of the ideas she is putting forward are purely theory, but she welcomed other scientists to engage in conversation about it over a beverage! Conferences like this one are a fantastic place to exchange ideas.
Rgenta Therapeutics: oral drugs targeting DNA repair
The final talk of the day came from Travis Wager of Rgenta Therapeutics. Travis told us about drugs his team is developing which can be taken by mouth to target the modifier PMS1, with the aim of slowing down somatic expansion in human clinical trials.
Rgenta has spent the last 4 years thinking about how they can go after targets that have traditionally been considered “undruggable”. Others have tried to target genes that control somatic expansion, like PMS1 and MSH3, in the past without success. Rgenta’s drugs act like glues that create a stronger bond between disease-causing RNA messages and the proteins that bind to them, in order to change how proteins are made, or to get rid of a faulty message or protein.
Travis highlighted the various diseases that they’re trying to target with their drugs against genes that control somatic expansion. This includes HD, myotonic dystrophy, Fragile X, and Fredreich’s ataxia: all repeat expansion diseases. Rgenta’s interest in HD is driven by the availability of huge amounts of data from both humans and animals, a testament to the collaboration and dedication of scientists and HD families. The HD field draws many companies for this reason.
Rgenta has targeted PMS1, because higher levels of PMS1 in people are associated with earlier HD symptom onset. Using mice, they’ve shown that changing levels of PMS1 seems safe and isn’t associated with cancer. Rgenta tested thousands of different chemicals known to change levels of RNAs, then measured which ones were best at changing the levels of PMS1, their target. So cool!
Their drug molecule that targets PMS1 is something called a “splice modulator.” It works by altering the PMS1 message molecule, which results in lower levels of the PMS1 protein. They’ve shown their drug works in lots of different cell and animal models. They then tested their PMS1-targeting drug in HD cells grown in a dish. In theory, lowering PMS1 should reduce somatic expansion. And that’s exactly what they found! Lowering PMS1 by about 90% essentially halted somatic expansion.
Rgenta’s compound also appears to only really target PMS1 levels without affecting other proteins. They also shared data which suggests that the compound works well in animal models, and does a good job getting into the brain. Right now Rgenta only has data showing that lowering PMS1 reduces somatic expansions in HD cells in a dish. But they’re marching forward with this approach, planning to test their drug in other models of HD. However, this is exciting news as it means there is another promising drug in the pipeline which might one day make it into the clinic if the animal studies continue to look good.
We are very happy to end Day 1 after reporting on so many cool things happening in labs at universities and companies around the world! Stay tuned for Day 2, coming shortly!
For more information about our disclosure policy see our FAQ…