Does a 'rusty hinge' cause Huntington's disease?
Canadian scientists have found that the mutant huntingtin protein is less flexible than normal.
By Professor Ed Wild August 27, 2013 Edited by Dr Jeff Carroll
Figuring out how the mutant huntingtin protein causes damage is the central problem of Huntington’s disease research. Now a team of Canadian researchers led by Dr Ray Truant has shown that the protein has an important ‘hinge’ function, which works less well in cells with the HD mutation. Exciting stuff, but contrary to what you might have read, it doesn’t mean we no longer need to study mice!
Huntingtin

A major advantage to researchers studying Huntington’s disease is that, unlike in many other brain diseases, we know exactly what causes it. A mutation in a single gene tells cells to make a harmful protein: mutant huntingtin. It’s mutant huntingtin that damages neurons and other cells, causing the symptoms of HD.
In the 20 years since its discovery, we’ve figured out a lot about what the huntingtin protein does normally, and what goes wrong when its toxic twin, mutant huntingtin, is made.
Studying huntingtin is crucial for our efforts to develop treatments for HD, because figuring out how and why mutant huntingtin causes harm is an important step towards preventing that harm.
Too many glutamines
Proteins are made from long strings of building blocks, called amino acids. These are connected like a string of beads, and the exact order of amino acids determines the shape and behaviour of the protein.
A small change in the order of amino acids can cause a big change in a protein’s shape, and that can dramatically alter how it does its job in cells, or even make it poisonous.
Mutant huntingtin is different from the ‘normal’ protein in just one way: near the beginning of the string, huntingtin protein has a sequence where one building block, glutamine, is repeated several times. The usual number of glutamines is between ten and twenty.
Huntington’s disease happens when there are too many glutamines.
What’s the magic number?
So, how many glutamines does it take before the huntingtin protein starts to cause harm? Curiously, the answer is thirty-seven. Below this, the protein huntingtin doesn’t cause Huntington’s disease as far as we know. Above it, HD is inevitable, as long as a person lives long enough.
Why on earth would things change at 37 glutamines? We don’t know! But unravelling this mystery is a top priority for HD researchers.
Even more strangely, while most people with Huntington’s disease have between 40 and 60 glutamines, that number of extra glutamines doesn’t produce disease in other animals, like mice. Animal models of HD need to have well over a hundred glutamines in their huntingtin protein before we start to see symptoms.
This is another mystery, and one that suggests that huntingtin causes subtle but very important chemical problems in humans, which our animal models can’t detect.
What about CAG?
“Human cells could be used to identify drugs that help the hinge to work, but they’ll still need to be tested in animals, including mice ”
Where do these extra glutamines come from, you may be asking. The answer lies in the HD gene, which is a recipe or set of instructions for making the huntingtin protein. Just as the protein has lots of glutamines near the beginning, the gene has a stretch where the chemical ‘letters’ C-A-G are repeated. The number of ‘CAG’ repeats in the gene corresponds to the number of glutamine building blocks in the protein. And if there are too many CAGs in the gene, there’ll be too many glutamines in the huntingtin protein.
So what’s new?
Professor Ray Truant, his student Nick Caron and Truant’s team at McMaster University in Canada have just published an article in the journal PNAS, describing their work to study how the number of glutamines affects the huntingtin protein. For the first time, they’ve found a potentially important change that happens around the critical 37-glutamine number.
The stretches of protein on either side of all those glutamines are bits called the ‘upstream’ and ‘downstream’ regions. Previous research has suggested that each of these plays a part in giving the mutant protein its harmful properties.
What Truant’s team did was ask whether the upstream and downstream regions might actually be working together, with the glutamine bit in between acting like a hinge. They also wondered whether his ‘hinge’ might not work properly in the mutant protein.
Time for a Lord of the Rings analogy
To investigate this, they needed a way of figuring out whether the upstream and downstream regions ended up close enough to work together. They used a clever kind of ‘proximity sensor’ called FLIM-FRET.
Remember Frodo’s sword ‘Sting’ in the Lord of the Rings movies, and how it glows blue when orcs are nearby? FLIM-FRET is kind of like that.
The researchers stuck special light-sensitive attachments onto the huntingtin protein, one on the upstream region and one on the downstream regions. These do nothing unless the protein folds over, bringing the two regions together. When that occurs, light gets passed from one tag to the other, shines out (like Frodo’s sword) and can be measured.
The rusty hinge of HD
Truant’s Sting-like proximity sensor proved that the upstream and downstream regions do end up folded back together in cells, with the glutamine stretch acting like a hinge. What’s more, it glowed less brightly when there were 37 or more glutamines in the huntingtin, suggesting that problems with the hinge begin around this number. Truant calls this the ‘rusty hinge hypothesis’ and suggests it’s one way that the mutant protein might cause harm.
Truant’s team confirmed these findings using proteins of several different lengths and ultimately showed the same abnormality in skin cells taken directly from a Huntington’s disease patient.
Tying it in
How does this ‘rusty hinge’ idea fit in with other things we already know about mutant huntingtin?
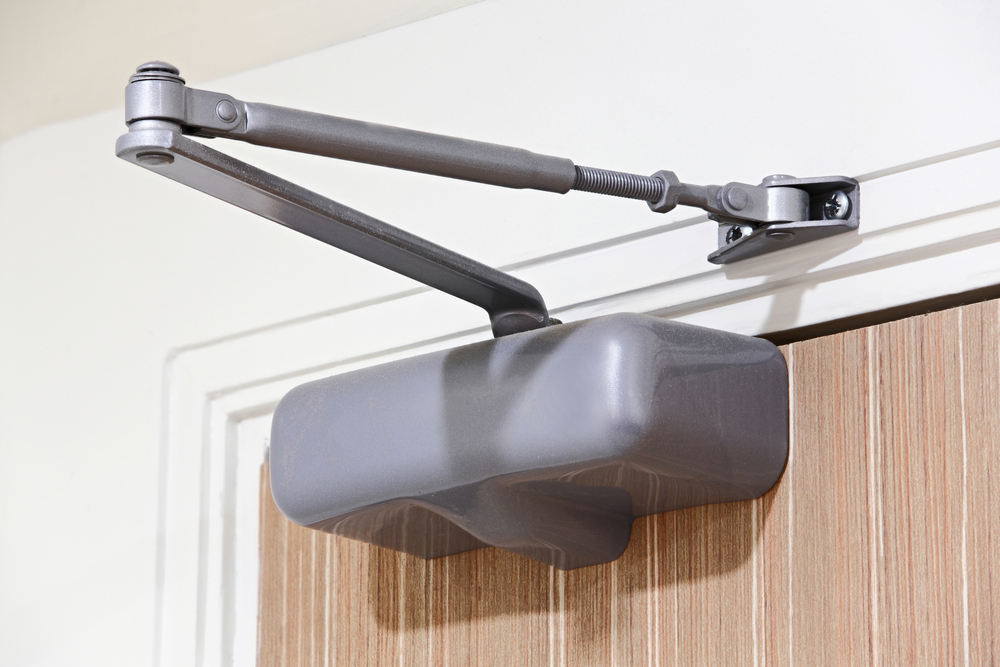
Well, we know that another protein called PACSIN can stick to huntingtin’s downstream region. Here, Truant’s team showed that it can also stick to the upstream region, suggesting it might be able to hold the two regions together across the glutamine hinge - like a door-closing mechanism.
When PACSIN was artificially removed, the proximity-sensor glowed less, suggesting that the upstream and downstream regions had sprung apart. Changing the behavior of PACSIN could overcome the hinge problem - a possible new approach to think about treating Huntington’s disease.
The other link to our existing understanding comes from tiny chemical changes that cells make to proteins. These chemical ‘tags’ can change how proteins behave and where they end up. Truant’s team had previously shown that ‘phospho’ tags are important for determining where the huntingtin protein goes and how harmful it is.
In their latest work, they demonstrated that these phospho tags can also have an effect on the activity of the glutamine hinge. This provides further support for the idea that drugs to control phospho-tagging might be able to prevent some harmful effects of the mutant protein.
What this research shows
Truant’s team has done really nice work, inventing a proximity sensor (or borrowing one from Frodo Baggins) and using it to show that huntingtin’s crucial glutamine region - the bit that’s too long in HD - functions as a hinge, allowing the upstream and downstream regions to work together.
Meanwhile another protein, PACSIN, spans the hinge and pulls them together. And they’ve linked the hinge function to the phospho-tagging of huntingtin. What’s more, this hinge property seems to start going wrong with roughly the same number of glutamines that cause disease in human patients.
Not only is this new information about the huntingtin protein, and how it causes damage. It also gives us new ideas of possible treatments - by changing the behaviour of PACSIN1 or the machinery that controls phospho-tagging.
What it doesn’t show
This wouldn’t be an HDBuzz article without a note of caution. In this case, we think it’s important to highlight some of the things the research doesn’t tell us - contrary to what you might have read online.
CBC news reported that the work “bypasses the need for mouse trials”, because Truant’s findings were able to be seen in human cells. Unfortunately, that’s not true. Human cells could be used to quickly identify drugs that help the ‘hinge’ to work better, but those drugs will still need to be tested in animals, including mice. To beat HD, we’ll need all the help we can get. No single animal model is perfect for developing drugs - but neither are human cells growing in a dish, or even human patients on their own. Different model systems each contribute useful information to help us to make progress.
Another thing this work doesn’t give us is a complete understanding of how the hinge problem causes the huntingtin protein to become harmful. A lot of the time in research, behind every door we find another door, which takes more work to open. Truant’s team and others will now work on understanding the bigger picture of how huntingtin’s hinge problem affects the workings of the cell and the brain. And turning these findings into treatments that could be taken by patients will take more work still. So, we keep going!