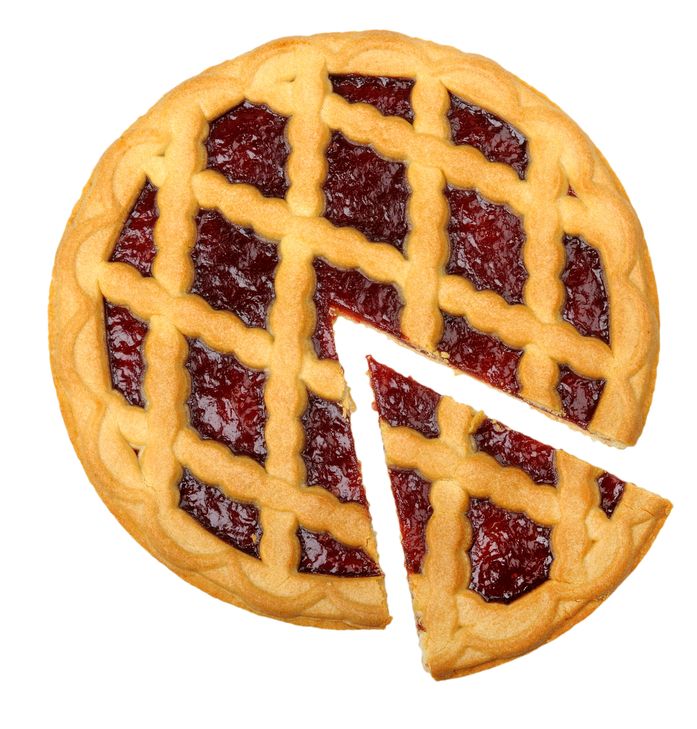
Splicing with danger: a new way of thinking about the harmful Huntington's disease protein
Researchers discover a new way harmful fragments of the huntingtin protein are made in neurons.
Researchers are hard at work figuring out exactly how the expanded Huntington’s disease gene causes harm. Recent work from a UK group has uncovered another clue to help solve the mystery. It turns out that faulty processing of the huntingtin ‘recipe’ produces a short, harmful fragment of the huntingtin protein.
The cookbook, the recipe, and the cherry pie
Huntington’s disease is caused by an unwanted expansion of the huntingtin gene. But genes are made of DNA, and it’s the expanded huntingtin protein that causes the problem. How do we get from DNA to protein? Via an intermediate messenger molecule called RNA.
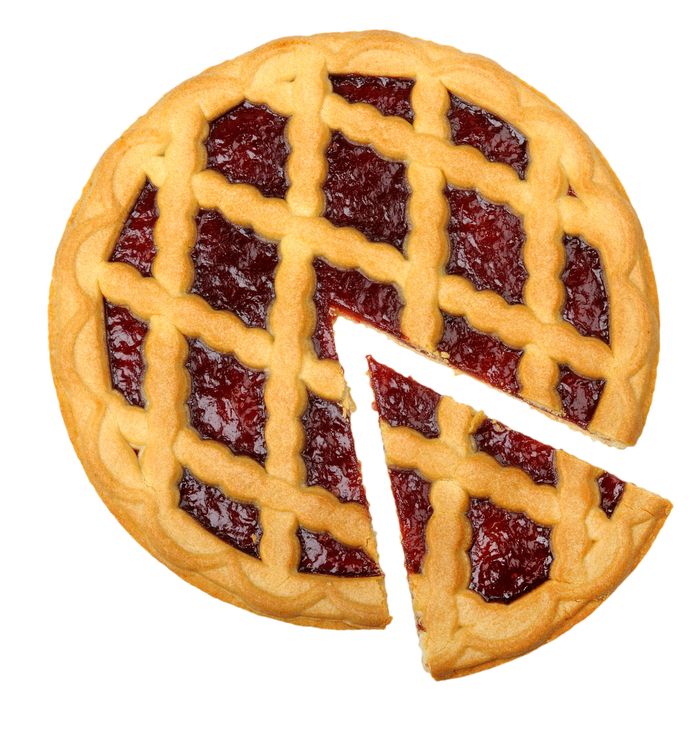
It may help to imagine an overzealous recipe-guarding grandmother who keeps her cookbook locked in a vault so that it doesn’t get damaged in the kitchen. Anyone who wants to make her famous cherry pie must go into the vault, make a photocopy of the recipe, and go out to the kitchen to assemble the ingredients.
In much the same way, our cells guard our DNA in the cell nucleus. RNA copies of genes are made in the nucleus, and transported out, where they are “translated” into protein. RNA messages act like recipes telling the cell exactly what ingredients to use to make the protein.
In the case of an expanded huntingtin gene, the RNA copy of the recipe is also expanded. The final protein has too many “ingredients” and isn’t formed properly. Although we know that this expansion causes Huntington’s disease, it is still not understood exactly how the expanded protein causes trouble in neurons.
The long and short of it
The huntingtin gene is very long – one of the longest genes we have – and stores the recipe for a very big protein. But the abnormal expanded region is right at the very start of the gene: the first line of the recipe, if you will.
One thing that researchers have noticed is that the brain cells of HD patients and mouse models contain very short versions of the huntingtin protein – only the first five per cent or so.
So how do these fragments come to be? Up until now, it was understood that special ‘cleaver’ proteins slice up the huntingtin protein, fragments of huntingtin.
Fragments that contain the abnormal expansion, though, are harmful to brain cells. Researchers led by Prof Gill Bates of King’s College London suggested that there’s another possible way these fragments could come about, and it occurs at the stage when the RNA copy of the recipe is made.
The cutting room floor
Recall that genes are made of DNA, which is copied into RNA, which is then translated into protein. Simple, right? But as with most things in nature, there is another layer of complexity to consider.
“Splicing, where non-coding gibberish is removed from the RNA message, goes wrong in Huntington’s disease.”
In fact, genes contain coding and non-coding regions that are arranged in sequence like a zebra’s stripes. Only the coding regions of the gene end up as protein, while the non-coding regions are skipped out.
So in the process of copying the DNA into RNA, first a copy is made of the entire gene, and then the non-coding regions are removed from RNA, in a process called splicing.
If we refer to our grandmother’s cookbook analogy, we can imagine that the cookbook has lines of gibberish inserted amongst the instructions. The whole recipe, with gibberish included, gets photocopied inside the vault, but the copy is cut up and stuck back together, without the gibberish, before going out to the kitchen.
So what’s new?
Studying mice, Bates’ team has found that the splicing step, where the non-coding gibberish is removed from the RNA message, goes wrong if the huntingtin RNA is expanded, as it is in Huntington’s disease.
In normal mice, the non-coding region was spliced out properly and the first two coding regions were joined together correctly to form a sensible, full-length message.
But in mice engineered to carry an expanded huntingtin gene, the first non-coding region was not removed properly. Within this gibberish region lies a signal telling the cell to “cut this RNA short”. As a result, mice with an expanded HD gene produce an extra, short RNA message made of just the first coding region and part of the non-coding region.
Once this short RNA message is translated into protein, the mice end up with a short fragment of the huntingtin protein, containing the expanded region: the very same short fragment that’s thought to be harmful in HD.
The team looked at samples from human Huntington’s disease patients. The abnormally short RNA message and protein were found in some, but not all of them. That may be because the production of the small fragments varies between different body regions or between patients.
How does the expansion in the RNA copy mess up the splicing process? Bates’ team showed that a protein usually responsible for the editing of RNA message molecules actually sticks to expanded huntingtin RNA, but not to normal huntingtin RNA. It may be that this inappropriate sticking interferes with proper splicing, resulting in the faulty short RNA copy of huntingtin.
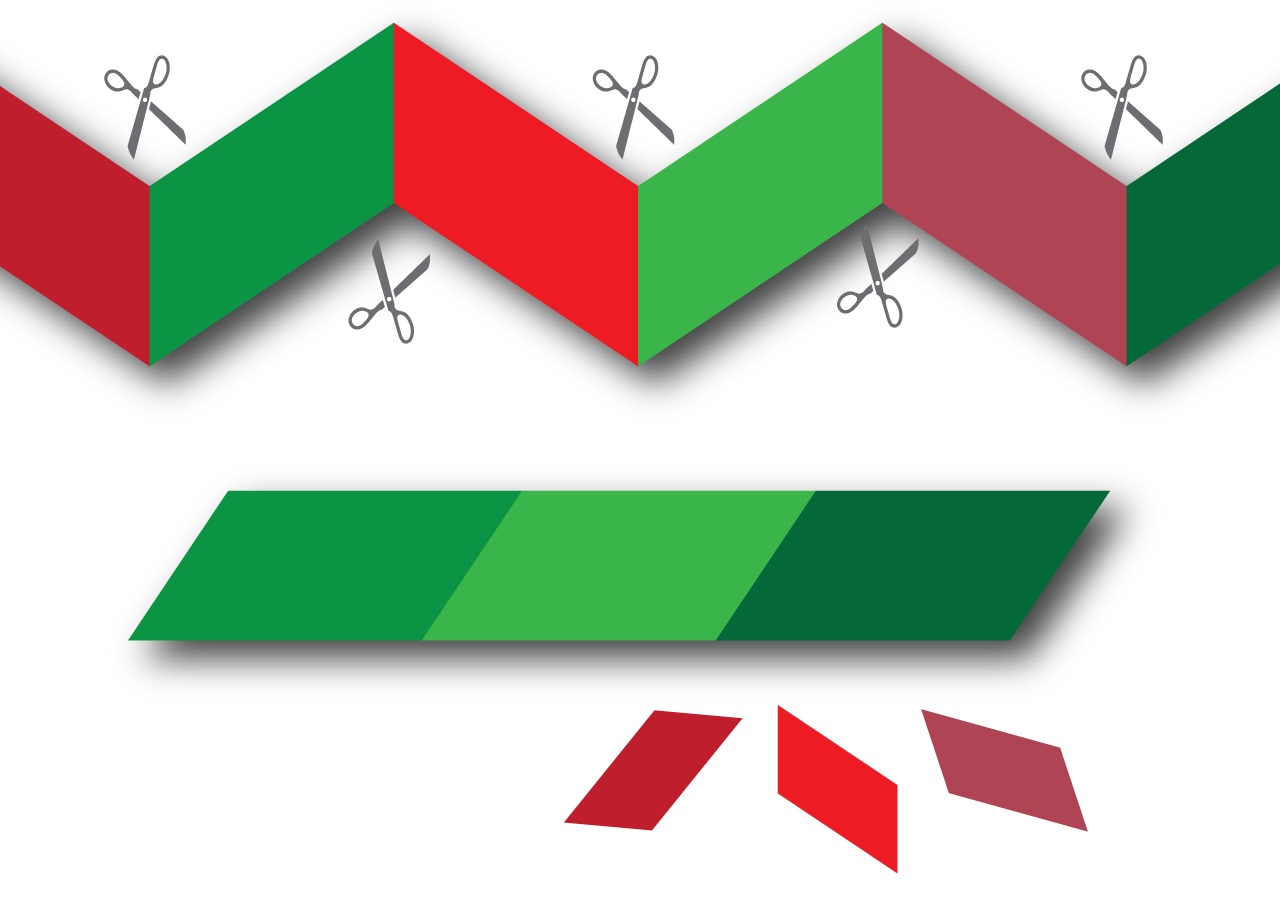
What do we do with this clue?
This study helps us to understand a new possible way in which harmful fragments of the huntingtin protein are generated.
Our brains and neurons are complex things, and this new mechanism may not be the only way through which harmful huntingtin fragments come about. The traditional ‘cleaver’ mechanism isn’t ruled out by this new finding, and in fact both mechanisms may be happening at once.
What’s more, harmful fragments are probably not the only way the expanded huntingtin protein does its damage.
But this new information is an important addition to our knowledge of how expanded huntingtin behaves in the brain. And the more we know, the better equipped we are to tackle the problem.
One possible implication of this work is for so-called ‘gene-silencing’ therapies for Huntington’s disease, which aim to reduce production of the huntingtin protein, by sticking to its RNA message molecules and telling cells to get rid of them.
Until now, it was thought that all the huntingtin RNA in the cell was the full-length version. Researchers will have to bear in mind that some of the detrimental huntingtin protein may come from a shorter RNA message, which may be missed by some gene-silencing drugs.
Thankfully, since we’ve already seen gene-silencing drugs work in several animal models of HD, it’s clear that this new research doesn’t invalidate that approach. In fact, through improving our understanding, it gives us new ways of understanding how the HD gene causes Huntington’s disease, and adds ‘abnormal splicing’ to our list of possible targets for solving the problem.
Learn more
For more information about our disclosure policy see our FAQ…