Gone fishing: protein network screen identifies new therapeutic targets in Huntington's disease
'Discovery-driven' screen reveals new protein networks involved in Huntington's disease damage & new drug targets
By Carly Desmond January 18, 2013 Edited by Professor Ed Wild
The mutant huntingtin protein doesn’t do damage in isolation - all proteins work in connected networks. Researchers at the California Buck Institute for Research on Aging have conducted a large-scale screen to identify protein networks that may be acting to relieve or worsen the harmful effects of the Huntington’s disease mutation. Could manipulating these networks offer new therapeutic options for HD?
The Huntingtin Protein: Getting to the Root of the Matter
2013 marks the 20th anniversary of the discovery of the genetic cause of Huntington’s disease. For the first time, scientists learned that a repeating DNA sequence in single gene was abnormally long in people who develop HD. Each of our genes provides the instructions our cells need to make a particular protein, which in the case of the HD gene is a protein we call huntingtin. Mutation of this genetic instruction set causes the huntingtin protein to be built with a mistake, leading to subtle changes in the protein’s cellular behaviour. As a person with HD ages, these changes have serious consequences, particularly within the neurons of the brain.
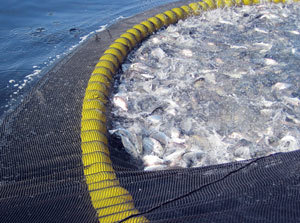
This ground-breaking discovery made it a possible for scientists to focus their efforts on a very specific task; in order to truly understand the disease, they would need to learn all they could about the huntingtin protein itself. This means knowing not only what the huntingtin protein does in the body of a healthy individual, but also what is going wrong in the disease.
What could be so important about one protein?
Proteins are often referred to as having specific ‘functions’ in the cell. To understand what this means, it may be helpful to imagine each cell in our body as a busy factory. It takes a lot of different people doing many different jobs to keep the operations of a factory running smoothly. All of these employees have a specific set of skills, and if any one person fails to do their job properly, the productivity of the whole factory is at risk.
Well, if our cells are like factories, proteins would be the employees. Just like the individual workers, each protein has to do a set of jobs, or ‘functions’. When the huntingtin protein is mutated in HD, it affects the way huntingtin does its functions.
Just as no one person could do every job in the factory, no one protein works completely alone. Instead, it exists as part of a network of proteins that interact with each other to work efficiently as a team. So to understand a protein’s function, scientists they must also determine what relationship it has to other proteins in the cell.
It has been estimated that our DNA provides the instructions to construct over 30,000 different proteins. Understanding how all of these proteins are connected to each other, and how they might be affected in HD, becomes an almost overwhelming challenge.
Hypothesis and discovery
To help deal with the complexity of modern biology, some researchers have shifted from traditional ‘hypothesis-driven’ research to an approach called ‘discovery-driven’ research.
A hypothesis is a prediction that a scientist makes based on what they already know. A good hypothesis is one that can be easily tested. Here is a simple example: say we hypothesize that cats prefer eating chicken over tuna A way of testing this might be to put out two bowls, one containing each choice. By counting the number of different cats that approach each bowl, evidence will be provided to either support or reject the hypothesis.
Hypothesis-driven research works really well, provided you already know a fair amount about the particular thing you are investigating. However, when aiming to figure out what a protein does in a network of thousands of other different proteins, progress can go pretty slow when asking only one question at a time. Imagine you wanted to find out which was the cats’ favourite out of 30,000 foods - but could only test them two at a time!
Discovery-driven research is a way to highlight biological processes that might be involved in a disease. You could say this approach generates not answers, but better questions. It tells researchers where to focus their attention for future studies.
Discovery-driven experiments, called screens, involve thousands of different mini-experiments conducted simultaneously.
“Some of the networks were already known from previous studies, but some hadn’t been implicated in HD before. ”
In some ways, biological screens are a bit like the difference between fishing with a trawling net instead of a rod and line. It’s a powerful technique, but requires more effort to sort through what’s caught.
Silencing individual genes with RNAi
A new study published in the journal PLOS Genetics, headed by Dr. Robert Hughes of the California Buck Institute for Research on Aging, describes a discovery-driven screen looking for protein networks that are affected by mutant huntingtin. Using a technology called RNAi, Hughes’ team worked to identify individual proteins that might be contributing to the harmful effects of the mutant protein.
RNAi stands for RNA interference, and is a form of ‘gene silencing’. RNAi is used to reduce the level of a single protein in the cell. This helps to determine what that protein might do and its importance to other cellular activities.
RNAi intercepts the chemical message that’s produced when a protein is being made, and destroys it - preventing the protein from being built. Virtually any gene and its corresponding protein can be targeted using RNAi.
An RNAi screen and some toxic fragments
First, Hughes’ team grew cells in the lab that had been genetically modified to make them produce the most damaging part of the mutant huntingtin protein. This mutant huntingtin ‘fragment’ causes the cells to die more quickly if the proper nutrients are not available. The health of the cells can be assessed by measuring changes that occur when a cell is dying.
To identify proteins involved in mutant huntingtin’s harmful effects, Dr. Hughes and colleagues used a ‘library’ of over 7,000 RNAi chemicals, each one targeting a different protein.
Those 7,000 RNAi chemicals were each tested on a separate batch of cells. In this way, the researchers were able to analyze the effects of each protein that was ‘switched off’. If silencing a gene makes the cells die more quickly, it suggests that the corresponding protein might normally be protecting the cell. And if the cells die more slowly, it means the protein might be worsening things in HD.
Crunching the numbers
Experiments like this produce a ton of data, so computers used to analyze and make sense of it. Thankfully, many protein networks have already been mapped out using more traditional scientific approaches.
The computer creates a new map, placing the ‘hits’ from the new data onto the existing network map. Using this technique, Hughes’ team found some networks that had more hits than expected, indicating that they might be important to the development of Huntington’s disease.
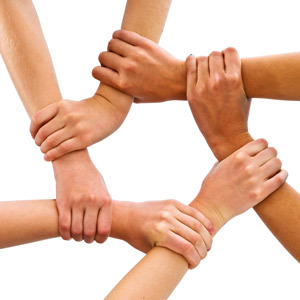
Some of these networks were already known from previous studies, giving the scientists confidence that their discovery-based approach was working. However, they also came across networks that hadn’t been implicated in HD before. One network in particular, connected to huntingtin through a protein called RRAS, was highlighted by the screen.
Because of the huge numbers involved, it’s important to do separate follow-up experiments to verify the most striking findings. So Hughes’ team did experiments in several different cell models, as well as in HD fruit-fly model, and found that RRAS was able to protect against cell death. Even better, they were able to pinpoint specific activities of the proteins within the network that might be easiest to target with drugs.
Knowing our limits
The most exciting aspect of this study is that it highlighted new networks that may be involved in Huntington’s disease. However, just as there’s no fishing net big enough to trawl the entire ocean, some important protein networks were likely missed with this work.
One reason for this is the cell model used in the screen. Instead of making cells produce the full-length mutant huntingtin gene, the researchers opted to use only a small fragment. That means that any proteins or networks that rely on the full huntingtin protein will have been missed.
Another reason is the cell type used in the experiments. This work was done with commercially-available cells called HEK293. These cells are easy to grow in big batches for large scale experiments like this. But after being altered to have such easy-going properties, they no longer behave the same as a normal, healthy cell in the body - and are certainly very different from neurons.
To make up for these potential weaknesses in the experimental model of the initial screen, all of the RNAi ‘hits’ were re-tested in cell lines that produce full-length huntingtin, as well as more complex fruit-fly models of the disease. And the RRAS network was examined in an HD mouse model.
What’s next?
This work represents a major effort on the part of the researchers involved. Biological screens require a lot of careful planning! However, with this study under their belt, the team could continue this work by performing a similar screen in cells containing full-length huntingtin.
When it comes to the hits of the current screen, there is still so much more to explore. One approach might be to investigate the RRAS network - or even repeat the screen - in more ‘accurate’ cell models, such as stem cells generated from real HD patients.
Whatever the future of this research holds, this is a good example of how discovery-driven research can generate new targets and ideas, provided we remain aware of the limitations of the techniques. We look forward to learning more about how these new protein networks influence the development of HD, as well as how they might be manipulated in the search for treatments.