Knockouts for the win: how expanding CAGs drive disease
A recent paper links CAG repeat expansion with widespread RNA changes, especially in brain cells vulnerable to HD. “Knocking out” certain DNA repair genes that go awry in HD can have positive effects on features of HD in mice.
By Dr Leora Fox April 14, 2025 Edited by Dr Rachel Harding
Huntington’s disease (HD) is caused by extra repeats of the DNA letters CAG within the genetic code of the huntingtin gene. We used to think that those CAG lengths were stable in most tissues, but we now have a growing understanding that CAG instability contributes to HD. Somatic instability is the concept that CAG repeats expand over time in some types of cells, particularly cells that are vulnerable in HD. Many scientists think this process might play a role in speeding up the development of symptoms.
A current paper delves into some of the machinery behind this phenomenon, asking how CAG expansion is connected with disease, and how science could harness DNA repair genes as a treatment for HD.
From people to animals… and back to people

Our current understanding of the phenomenon of CAG repeat expansion stems from huge human studies in which participants with HD donated samples and clinical data. Their contributions enabled scientists to link subtle differences in people’s genetics with the age when they developed HD symptoms. These studies, known as genome-wide association studies, or GWAS, (pronounced ‘gee-wass’) showed that certain DNA variations could greatly hasten or delay the onset of HD.
Many of the genes identified in these studies that warranted further research belong to a family of genes that help repair DNA. The past few years have seen a flurry of activity leading us to some new conclusions about HD and expansion of CAGs. Here’s a brief recap before we dive into some novel data around this topic:
The DNA repair machinery can slip up when trying to “correct” extra-long CAG repeats - accidentally making them longer and longer!
This does not happen in the vast majority of cells, but seems to occur a lot in a part of the brain called the striatum, which controls mood, movement, and motivation. Studying CAG expansion in the striatum could lead us closer to understanding why these cells are so vulnerable in HD.
Some studies have found that there is a threshold of around 150 CAG repeats at which damage to the cell starts to speed up.
Experimental “knockout” or genetic removal of DNA repair genes known to make mistakes on CAGs can slow down or even stop CAG repeat expansion in lab models of HD.
Some of these genes, like Msh3, are the targets of human HD therapies in development - but we still need to understand more about how they influence HD biology, and what are the consequences (positive and negative) of knocking them out.
Setting up the experiment
“One way in which this work represents a step forward is that the authors show how knockout of the genes that prevent CAG expansion can also have effects on brain cells and mouse movement. ”
The authors of a recent paper, led by X. William Yang at The University of California, Los Angeles (UCLA), took a direct and thorough approach to exploring the connection between DNA repair genes, CAG expansion, brain cell health, and even behavior.
They chose a set of nine genes identified in the human GWAS studies and which form part of the machinery that performs a certain type of DNA repair, the kind that drives the “oops” of CAG lengthening. Then they made use of specialized mouse genetics and breeding schemes to create HD mice missing one or both copies of these DNA repair genes.
In each of these HD mice, missing genes like Msh3, Pms1, Mlh1, and others, they could learn more about how messing with DNA repair might affect CAG expansion, RNA message production (the lab’s specialty), toxic huntingtin buildup, and other features of HD. They were surprised to learn that some knockouts had profound positive consequences, whilst others had no effect at all. What they learned is valuable for our understanding of HD biology and the development of therapeutics.
Reversing RNA changes
Our DNA is read or “transcribed” by specialized machinery to make RNA messages, which are eventually used to make proteins, the building blocks of life. There is a whole branch of science that explores the location and amount of RNA message made from different genes - this is the field of transcriptomics.
Scientists can define a healthy mouse “transcriptome” by looking at thousands of genes and asking which are normally turned on and off in different cells, and how much of each RNA message is present. Then they can look at how this changes in an HD mouse over time, or experiment to see what might help restore the mouse’s RNA levels to normal.
The Yang lab was working with one type of mouse that models HD that shows major changes in its transcriptome compared to regular mice. Lots of genes are producing more or less RNA than they are meant to, especially within medium spiny neurons, the cells that are most vulnerable in HD. When the Yang lab “knocked out” half of the Msh3 and Pms1 in their HD mouse model, they saw a partial reversal of the RNA changes in medium spiny neurons. With Msh3 or Pms1 fully gone, the RNA changes were almost fully reversed, often lasting up to a year (half a lifetime for a lab mouse!). Knocking out a couple of other genes - Msh2 and Mlh1 - also had some reversal effects, but these were more moderate. Some gene knockouts had no effect at all.
Members of the Yang lab are world experts in studying HD transcriptomics, and they used multiple cutting-edge laboratory techniques as well as different statistical approaches to confirm their results. They examined RNA levels across many cells, down to the level of single cells, and also looked at how tightly the DNA was wound around its “spool,” known as chromatin. In all cases, knocking out Msh3 and Pms1 seemed to reverse the HD-related changes.
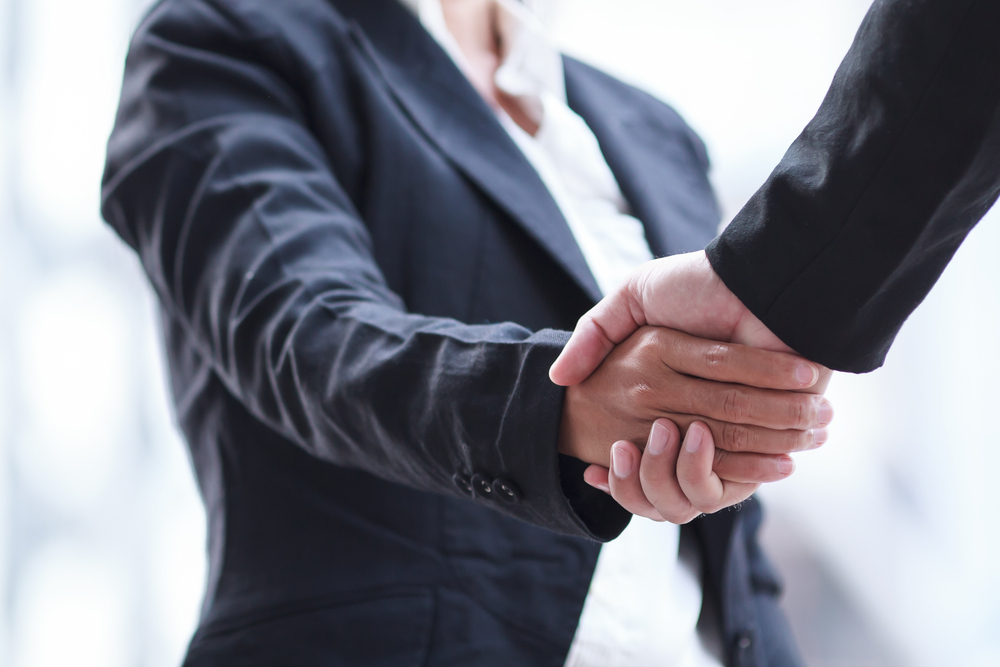
Calming CAGs and clumps
In parallel, the Yang lab measured the amount of somatic instability - the lengthening of CAG repeats - in different parts of the brain and body. In this type of HD mouse, CAG repeats get longer over time, especially within the cells of the striatum. In fact, this group used statistics to define the rate at which CAGs expand in these vulnerable mouse brain cells: it’s about 8.8 CAG repeats per month. (These rates of expansion do NOT apply to humans - these mice start out with 140 repeats and are designed for experimentation.)
The exciting finding is that when the mice had less or no Msh3 or Pms1, that rate went way down. In fact, removing both copies of Msh3 slowed that rate down to 0.3 extra CAG repeats per month, all the way up to 20 months old - that’s basically a stable repeat length, in an old mouse!
At the same time, Yang and colleagues observed that HD mice with half or no Msh3 or Pms1 also had far fewer clumps of huntingtin protein in the striatum. The buildup of these clumps is a classic feature of HD that many scientists suspect could be toxic to brain cells. Removing Msh3 prevented the formation of huntingtin clumps in other areas of the brain as well. The amount of clumped-up huntingtin seemed to correspond with the amount of abnormal RNA changes they’d previously seen.
Furthermore, they were able to confirm results from other labs showing that there seems to be a threshold of CAG repeats - around 150 - above which the cell begins to experience more stress. They connected this threshold to higher levels of RNA changes: CAG expansion speeds up and worsens that stress.
Preventing brain and behavioral changes
We love math and multi-panel rainbow graphs, but it’s even cooler to see a link between genetics and behavioral health. One way in which this work represents a step forward is that the authors show how knockout of the genes that prevent CAG expansion can also have effects on brain cells and mouse movement.
This type of HD mouse tends to show changes in the connections between neurons, known as synapses, as well as enlargement of support cells called astrocytes. The mice also have problems with their gait and movement. However, when the researchers knocked out Msh3, they no longer observed any of these HD-related changes in the mice. This is even further evidence for Msh3’s role in HD, and suggests that it is a good drug target.
“Publications like this one represent years of collaborative work among a large team, shaped by frequent input from an international community of HD scientists. ”
Note that this wasn’t a main focus of the paper - they only looked at a few features of brain health and one behavioral task - but it’s still a promising link.
Small steps power future treatments
You have likely noticed that HDBuzz has been harping on somatic instability (loudly and frequently) for a while now, and that we’ve presented lots of similar messaging: CAG repeat expansion seems to be contributing to HD, and scientists have identified some ways to combat it. This work is no exception; once again, genes like Msh3 and Pms1 are culprits that can be “knocked out” to great benefit in the brains of one type of HD mouse.
All these individual advances may appear to be small, but publications like this one represent years of collaborative work among a large team, shaped by frequent input from an international community of HD scientists. We chose to highlight this particular paper because it connects the dots between CAG expansion, abnormal RNA messages, and changes in brain health and behavior.
The authors caution that we need much more information to truly understand the link between Msh3 and Pms1 and the symptoms of HD. They also acknowledge, as we always do, that mice are not people. These mice in particular begin life with 140 CAGs in every cell of their body, which is much higher than even most cases of human juvenile HD. CAG repeats are not expanding anywhere near as quickly in humans as they do in these experimental mice.
Nevertheless, their data, along with that of other labs working tirelessly to understand HD, makes a strong argument for developing therapies based around Msh3 and Pms1. And these efforts are indeed under way!